UPDATE:
LIGHT DAMAGE TO THE EYE
NOV. 1997
Prolonged exposure to non-visible UV and infrared light can have damaging effects on the eyes. Here, we update current knowledge of this much-debated topic.
Human eyes respond visually to electromagnetic wavelengths from about 380 to 780 nanometers (nm). Longer infrared and shorter ultraviolet (UV) wavelengths do not elicit visual response, but they enter our eyes and may cause heating and photobiological damage. All electromagnetic wavelengths travel at the same speed in a vacuum, but they have differing energy levels; the shorter wavelength photons have more energy and potential to cause biological damage.
The UV spectrum (wavelengths from 200-380nm) is divided into three parts based upon potential biological effects. UV-A may be involved in development of brunescent cataracts, pinguecula and pterygia, while UV-B may contribute to photokeratitis, cortical cataracts and erythema. UV-C from the sun does not reach the Earth's surface because it is blocked by the stratospheric ozone layer. Less than 10 percent of the light energy from the sun that reaches our planet is UV, and 90 percent of this is UV-A.
The sun is the strongest source of light to which most of us are exposed, and the stratospheric ozone shield helps protect us from its more damaging wavelengths. Over the past several decades, man-made chemicals, especially the fully halogenated methanes (CFCs), have reduced the ozone shield, heightening concern about abiotic effects to our skin and eyes. The most dramatic example of ozone depletion is the Antarctic's "ozone hole." Since 1980, there has been a three to six percent per decade decrease of ozone in the northern hemisphere. However, international agreements over the past 10 years should lead to a phase-out of CFC and other chlorine-bearing chemicals by the year 2000, and over several decades this should restore the ozone layer.
BIOCHEMICAL MECHANISM OF LIGHT DAMAGE
To initiate photodamage, tissues must contain a molecule that absorbs light. Tissue damage may occur either through molecular bond breaking or creation of free radicals.
Molecular Bond Breaking
Proteins, enzymes and nucleic acids contain alternating double bonds, and UV wavelengths cause such molecular configurations to resonate. We can see the analogy with opera singers who break wineglasses by striking certain notes. They tap the glass to find its resonating frequency, then they sing that frequency as loudly as they can. The resonating frequency can be thought of as fitting snugly into the glass structure. The increased intensity, like a dynamite charge placed in a rock crevasse, breaks the molecular bonds. The new molecules may either induce inflammation or neoplasia or affect the immune system.
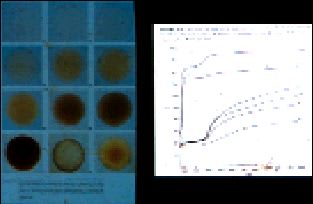
(From Lerman S., Photoxicity: clinical considerations, Focal Points 1987. American Academy of Ophthalmology San Francisco, CA)
Creation of Free Radicals
Pigmented molecules absorb specific wavelengths of visible and UV light, and this photon absorption ultimately changes the energy level to the unstable triplet state. The molecule then ejects an electron, which usually combines with a neighboring molecule. In cases of photodamage, the neighboring molecule is often oxygen. When the oxygen receives an extra electron, it is known as superoxide, one of a family of compounds called free radicals. Free radicals, or superoxidizers, can disrupt cell membranes, mitochondrial membranes and nucleic acids, as well as depolymerize collagen and hyaluronic acid, thereby destroying tissue.
Free radical light damage to tissue requires three components: a light absorbing molecule (dye, pigment), oxygen and short wavelength light. As a defense, there are molecules in the body that are constantly on the lookout to disarm any newly arrived free radical. These many scavenger molecules include the ubiquitous superoxide desmutase (SOD), Vitamin C, Vitamin E, glutathione peroxidase and carotene. Thus, a shortage of these scavengers can tip the balance toward greater light damage vulnerability. Therefore, we can say that UV radiation can be potentially damaging if a certain balance is upset.
GROUPS MORE VULNERABLE TO OCULAR LIGHT DAMAGE
There are several groups of people who are more vulnerable to ocular light damage: older people, lightly pigmented individuals, aphakics, people who use photosensitizing drugs, and people who have a problem with outer segment turnover.
Older individuals: The body has a series of protective molecules that either filter out the harmful wavelengths or scavenge the harmful photometabolites. With increased age, the concentration of some of these protective molecules may decrease. Age-related macular degeneration and cataract formation may be related to a combination of cumulative light exposure and a coincident decrease in protective biochemicals.
Lightly pigmented individuals: Studies have shown that people with blue irises (lighter pigmented eyes) have a significantly higher incidence of age-related macular degeneration than a control series of patients with brown irises. Accordingly, studies show that age-related macular degeneration is almost unknown in black African patients.
Aphakic patients: Some studies suggest that nuclear sclerosis in the elderly protects the retina from age-related degeneration. It has also been shown that the aging crystalline lens is an efficient filter against UV and blue light (Fig. 1). Retinal injury with short wavelength visible light has also been produced in aphakic monkeys. Absence of the crystalline lens, such as after cataract surgery, eliminates this natural filter of light, especially UV. Aphakics should compensate with appropriate UV blockers in eyeglasses or intraocular implants (IOLs).
People who use photosensitizing drugs: Chemical compounds with multiple cyclic rings containing alternating double bonds are often photosensitizing agents (Fig. 2). These agents can absorb UV and short wavelength visible light and then generate tissue-damaging free radicals. Compounds in this group include the phenothiazines, 8-methoxypsoralen (used to treat psoriasis), allopurinol, the tetracyclines and the hematoporphyrins used for phototherapy. When these compounds deposit in the lens or the retina, the tissues become more vulnerable to light damage.
Outer segment turnover: It appears that nature copes with the anticipated light damage to the photopigment-containing optic discs by having the retinal pigment epithelium digest a portion of the outer segment daily. If there is a malfunction in this digestive system due to genetics, malnutrition or injury, then there is a buildup of photoreceptor disc metabolites. Such a buildup is related to drusen deposition and clinical age-related macular degeneration. Thus, some form of faulty light damage defensive system may be, at least partially, responsible for age-related macular degeneration.
CLINICAL MANIFESTATIONS OF OCULAR LIGHT DAMAGE
Ocular structures that are prone to light damage include the eyelid, cornea, crystalline lens and retina.
Lids: The skin of the eyelid is subject to a multitude of light-induced changes including sunburn, which is basically a UV-B induced response. Clouds do not filter out UV light, so a cloudy day will not prevent sunburn. Because UV radiation is reflected off sand, water and painted surfaces, beach umbrellas cannot provide full protection from UV damage. More serious common skin conditions induced by UV radiation include actinic keratoses, age spots, skin dryness, wrinkling, sebaceous hyperplasia and comedones. If you don't believe that the sun exacerbates skin wrinkling, simply compare the sun-exposed skin of the eyelid to the protected skin of the abdomen of a 70-year-old individual. The most severe forms of UV-B induced damage are malignant skin changes including basal cell carcinoma, squamous cell carcinoma and probably malignant melanoma. In each of these, UV radiation has an impressive statistical correlation.
Cornea: Ultraviolet damage to the cornea is both wavelength and intensity dependent, and the UV-C group (260-290nm) comprises the most damaging range of wavelengths. Because the ozone layer prevents these wavelengths from penetrating to Earth, the sources of most actinic keratitis are man-made -- welding flashes, germicidal lamps and sun lamps. At 270nm, only 0.005mJ/cm2 of energy will produce a lesion. At 300nm, about 0.01mJ/cm2 of energy will produce a lesion. At 320nm, 10.5mJ/cm2 of energy, or an increase of 2,000 times above the lowest threshold, will produce a lesion. Thus, for short wavelengths, a very small amount of UV energy can produce a corneal lesion. The nucleic acids of the corneal epithelium maximally absorb these wavelengths, as do certain amino acids such as tryptophan. We should not forget that UV-B can also produce corneal lesions if the exposure is long enough. Snow blindness is a result of prolonged exposure to UV-B reflected from the snow, and fresh snow can reflect as much of 85 percent of the incident UV radiation. Clinically, actinic keratitis appears about eight to 12 hours after exposure. Pain probably arrives when the damaged epithelial cells desquamate, producing the characteristic punctate fluorescein staining. Chronic exposure to UV light is said to produce spheroidal degeneration of the cornea (Labrador keratitis and climatic droplet keratopathy). The incidence of this condition in the Eskimo population is about 14 percent. Epidemiologic evidence also suggests that chronic exposure to UV light produces or is associated with pterygium.
Lens: The chemical components of the crystalline lens are particularly vulnerable to different parts of the electromagnetic spectrum. For example, short wavelength ionizing radiation is cataractogenic, probably due to the creation of overwhelming numbers of damaging free radicals as well as molecular bond breaking. Several important epidemiologic studies show a connection between environmental levels of UV light and a higher incidence of cataract (primarily cortical) formation. Basic biochemical research gives further insight. Lens exposure to UV-A and UV-B leads to lens enzyme changes. Specifically, exposure of rat lenses to 5mW/cm2 of UV light (wavelength = 360nm) leads to almost total destruction of Na+ K+ ATPase activity in less than 24 hours. The lens of the grey squirrel is a favorite model because its yellow color is similar to that of the aging human lens. Exposing such lenses to 1.5 to 2mW/cm2 UV light (wavelength = 365nm) causes significant aggregation of insoluble lens proteins, which are responsible for the increased light scattering seen in the aging human lens and in human cataracts. Studies show that an inhibitor of singlet oxygen formation (sodium azide) completely prevents protein aggregation in the presence of UV light.
Infrared radiation can also affect the lens. The higher frequency (lower wavelengths) of infrared radiation closely match the resonant frequency of water molecules. Glassblower's cataract, in which the lens' water absorbs the radiation from the infrared source and literally cooks the surrounding proteins of the lens, has long been recognized as a clinical example of this relationship.
Finally, it's important to mention the relationship between age of onset of presbyopia and geographic latitude (Table 1). Presbyopia occurs about five years earlier in the tropics than in northern climates. Increased infrared from solar radiation has been implicated because in a specific latitude, the onset of presbyopia is earlier in coastal regions (less UV radiation) than in mountain regions.
Retina: There's no question that prolonged illumination from the indirect ophthalmoscope, the operating microscope or the sun can produce a level of light equivalent to the noonday sun at equinox and 40º latitude, which measures 12,800 foot candles. Light measurements from different operating microscopes show values from 5,120 foot candles to 28,160 foot candles at the surface of the patient's eye. Such intensity, directed on an eye with a dilated pupil for a prolonged period, can induce maculopathy. The illumination from the indirect ophthalmoscope with a focusing lens can produce a focal energy on the retina equivalent to that produced by the sun. We're not sure which specific wavelength emanating from these instruments produces the damage. It may be the effect of a combination of wavelengths, since photochemical damage from short wavelengths is enhanced by raising the retinal temperature by 3º-4ºC.
Age-related macular degeneration may be a manifestation of light toxicity. Experimental visible wavelengths (primarily blue light) have damaged monkey retinas. However, these experimental lesions do not resemble the clinical appearance of age-related macular degeneration in humans. We also know that bleached visual pigments (which end up as waste products such as lipofuscin in the retinal pigment epithelium) maximally absorb blue and UV-A wavelengths and that enhanced choroidal pigmentation seems to protect against this degeneration.
To complicate matters further, epidemiologic studies of age-related macular degeneration in the elderly are difficult to evaluate when a cataract is also present. A cataract can filter harmful light from the retina, but we don't know if the increased melanin works its protective effect as a light filter or as a metabolic agent. It would make sense that any condition in which bleached visual pigments build up in the retina might promote light damage. The increased choroidal melanin in black African patients might simply prevent the light reflected from the sclera from striking the vulnerable pigments on a second pass. We await further research in this area.
What is the role of light toxicity in retinopathy of prematurity (ROP)? Strong evidence associates the use of oxygen therapy with the development of the disease in premature infants. The combination of oxygen, light and the proper pigmented molecules can incubate damaging free radicals. Therefore, light may enhance the damaging potential of oxygen. Interestingly, fluorescent lights for nurseries were introduced at about the same time as oxygen therapy for premature infants. The production of a high concentration of free radicals in an immature retina (perhaps devoid of protective agents) could possibly lead to the disease. There are studies that might support the hypothesis that light damage is involved in ROP. Again, we await further research in this area.
TABLE 1 | ||||
---|---|---|---|---|
ONSET OF PRESBYOPIA IN DIFFERENT REGIONS |
||||
AGE | PLACE | LATITUDE | AUTHOR | YEAR PUBLISHED |
47 | England | 51-54 | Ayrshire | 1964 |
43 | England | 51-54 | Turner | 1958 |
45 | New York | 41-45 | Duane | 1912 |
50 | Cleveland | 38-42 | Allen | 1961 |
45 | Japan | 36-44 | Kajiura | 1965 |
43 | Japan | 36-44 | Fukuda | 1965 |
42 | Japan | 36-44 | Ishihara | 1919 |
43 | California | 32-42 | Hamasaki | 1956 |
45 | Texas | 26-36 | Fitch | 1971 |
41 | Israel | 32 | Raphael | 1961 |
40 | South Africa | 26-29 | Coates | 1955 |
37 | India | 10-30 | Rambo | 1960 |
39 | Puerto Rico | 18-18.5 | Miranda | 1977 |
LIGHT PROTECTION
The human eye is quite resistant to light damage. However, age, pigmentation, nutritional status, drops taken and genetic biochemical makeup can make some of us more vulnerable. In certain high illumination situations, wearing absorptive lens can improve visual function.
Improvement of contrast sensitivity: On a bright sunny day, irradiance from the sun ranges from 10,000 to 30,000 foot lamberts. These high light levels tend to saturate the retina and, therefore, decrease finer levels of contrast sensitivity. The major function of a dark sunglass (gray, green or brown) is to return the retina to a level of maximal contrast sensitivity. Most dark sunglasses absorb 70 percent to 80 percent of the incident light of all wavelengths.
Improvement of dark adaptation: Experiments have shown that if a person spends a full day at the beach on a sunny day and does not wear dark sunglasses, dark adaptation can be impaired for more than two days. Thus, dark sunglasses are recommended for prolonged periods in bright sun.
Reduction of glare sensitivity: A number of sunglass modalities can reduce glare sensitivity. Polarized sunglasses reduce the intensity of reflected light and dazzle glare from road surfaces, glass windows, lake and river surfaces, and metal surfaces. Since light reflected off a horizontal surface produces light polarized in the horizontal plane, properly oriented polarized sunglasses can eliminate this. Graded density sunglasses are deeply tinted at the top and gradually become lighter toward the center, removing dazzle glare sources above the line of sight. Wide temple sunglasses reduce glare sources from the side.
Improvement of color contrast: Orange sunglasses efficiently absorb wavelengths in the purple through blue/green range. All these colors appear as different forms of dark gray to the wearer. On the other hand, the wearer clearly sees the spectrum from green through yellow to orange to red. Colors appear slightly unreal, but color contrast improves. Patients with conditions such as cataracts or corneal edema, whose color contrast sensitivity has decreased, report improvements in color contrast with these sunglasses.
Photochromic lenses: These lenses are either glass or plastic (Fig. 3). When short wavelength light (300-400nm) interacts with glass photochromic lenses, they darken. The chemical reaction (the conversion of silver ions to elemental silver) is similar to the reaction that occurs when photographic film is exposed to light. However, the chemical reaction in photochromic lenses is reversible. With continued exposure to short wavelengths, the lens darkens to absorb about 80 percent of the incident light. When the illumination falls, the lenses lighten, absorbing about 20 percent of the incident light. These lenses take longer to lighten than darken. When darkened, photochromic lenses are also excellent ultraviolet absorbers. On the other hand, plastic photochromic lenses are coated with indolinospironaphthoxazine (ISN), an organic molecular compound that changes its shape and consequently its light absorptive properties when illuminated. ISN can be configured to turn different dark colors when illuminated.
Ultraviolet absorbing lenses: Almost all dark sunglasses absorb most of the incident ultraviolet light. Ophthalmic crown glass transmits all UV-A and a small amount of UV-B, while CR-39, a commonly used clear, hard resin plastic with a special coating, blocks all UV-B but transmits some UV-A. Polycarbonate plastic blocks all UV light. A study of the transmission curves for nine inexpensive clip-on sunglasses showed that all but the blue clip-ons blocked more than 90 percent of the UV. Figure 4 shows the absorption curve for clear glass, CR-39 and clear polycarbonate.
There are several contact lenses formulated with UV blockers, but their effectiveness is uncertain and they do not substitute for other UV protective eyewear.
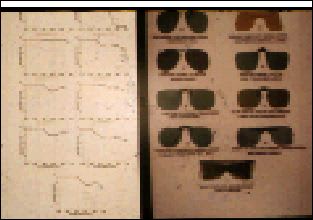
CONCLUSION
Most of us are not exposed frequently to prolonged excessive sunlight, and our eyes receive sufficient protection from dark sunglasses or photochromic lenses. People who are more vulnerable to ocular light damage such as older individuals, lightly pigmented people, aphakics and patients using photosensitizing drugs should take greater precautions. Although the present dimunition of the ozone layer has increased our concern and vigilance, it does not radically change our approach to ocular light protection. CLS
David Miller, M.D., is an associate clinical professor of ophthalmology at Harvard Medical School in Boston.
References are available from: Paul White, O.D., New England College of Optometry, 424 Beacon St., Boston, MA 02115.
Continuing Education
UV Update QUESTIONS
1. Human eyes respond visually to which wavelengths?
a. 200-350nm
b. 380-780 nm
c. 800-950nm
d. all of above
2. The ozone layer blocks out which of the following?
a. UV-A
b. UV-B
c. UV-C
d. None of above
3. About what percent of the sun's energy that reaches Earth is UV?
a. 10%
b. 20%
c. 30%
d. 40%
4. Since 1980, the per decade decrease of ozone in the northern hemisphere has been about:
a. 3-6%
b. 9-12%
c. 15-18%
d. 21-24%
5. Breaking the molecular bonds within tissues can initiate photodamage and induce:
a. inflammations
b. neoplasia
c. immune system effects
d. all of above
6. Superoxidizers, free radicals, can depolymerize:
a. mitochondrial membranes
b. nucleic acids
c. collagen
d. cell membranes
7. Age-related macular degeneration has the lowest incidence with native:
a. Norwegians
b. black Africans
c. Germans
d. Danes
8. Which of the following are photosensitizing drugs?
a. phenothiazines
b. allopurinol
c. tetracycline
d. all of above
9. Common skin conditions that may be induced by UV radiation include:
a. actinic keratoses
b. age spots
c. sebaceous hyperplasia
d. all of above
10. At 270nm, how much energy can produce a skin lesion?
a. 0.01 mJ/cm2
b. 0.005 mJ/cm2
c. 10.5 mJ/cm2
d. none of above
11. Snow blindness is a result of prolonged exposure to:
a. UV-A
b. UV-B
c. UV-C
d. none of above
12. Glassblower's cataract is caused by:
a. infrared
b. ultraviolet
c. visible light
d. microwave
13. Presbyopia occurs on average about how much earlier in the tropics than in northern climates?
a. 1 year
b. 3 years
c. 5 years
d. 10 years
14. Prolonged illumination from which of the following can produce maculopathy?
a. indirect ophthalmoscope
b. operating microscope
c. sun
d. all of above
15. Bleached visual pigments maximally absorb:
a. blue
b. UV-A
c. both "a" and "b"
d. neither "a" nor "b"
16. Retinopathy of Prematurity (ROP) may be due to:
a. oxygen
b. light
c. both "a" and "b"
d. neither "a" nor "b"
17. Dark sunglasses worn in the sun can:
a. improve contrast sensitivity
b. improve dark adaptation
c. absorb UV
d. all of above
18. Which of the following best reduces glare sensitivity?
a. polarized lenses
b. grey sunglasses
c. orange sunglasses
d. contact lenses
19. Orange sunglasses may improve color contrast for individuals with:
a. conjunctivitis
b. cataracts
c. detached retina
d. pterygia
20. Which wavelengths cause glass photochromic lenses to darken?
a. 300-400nm
b. 500-700nm
c. 800-1,000nm
d. 1,100-1,300nm