CORNEAL TOPOGRAPHY
Corneal Topography: The Evolving Technology
Here we look at the evolution, the current technology and the exciting future of this useful tool.
By Michael Twa, OD, PhD
![]() Dr. Twa is a clinician scientist by training. He received his clinical degree in Optometry from The University of California at Berkeley in 1990 and joined the faculty at The University of Houston College of Optometry in the Fall of 2007 after completing a PhD in Vision Science at The Ohio State University in 2006. He is an experienced clinician with expertise in refractive surgery and anterior segment disease acquired from clinical practice in the Department of Ophthalmology at the University of California, San Diego. |
The development of clinical tools for measuring corneal shape and understanding its impact on vision has a long history tied to early adoption of new technologies. There have been several recent developments that promise to greatly extend our ability to image and understand this most important ocular structure. Before we look forward, let's take a brief look back at where we have been and review how we arrived at where we are today.
In the 1950s and 1960s, pioneers in optometry were pushing the boundaries of corneal shape measurements by using profile projection and instant photographic techniques to quantitatively describe the cornea. At that time, contact lenses were an emerging technology and to successfully apply these new devices to the eye, we needed to know more about corneal shape.
In the 1970s and 1980s, two important technological advances pushed corneal imaging to another level: refractive surgery gained a toehold in ophthalmology, and personal computers became a reality. Refractive surgery created a new and urgent need for clinicians to know the shape of the cornea, how it responded to surgical manipulation and why changes in shape did not always produce the intended changes in vision.
At this same time, powerful, affordable microprocessors became widely accessible and by the late 1980s, quantitative representations of the corneal surface became a practical reality.
In the late 1990s, refractive surgery was well established worldwide as a legitimate specialty. The precision of excimer lasers further underscored the need to understand corneal shape and its impact on visual function. The first scanning slit-based corneal topography systems soon came to market, enabling clinicians to look at both anterior and posterior corneal surfaces. These systems also introduced practitioners to elevation surface maps that complemented traditional curvature displays of corneal shape data.
Today's Topographers
The pace of technological developments in corneal topography has not slowed, and today's corneal topographers are more powerful than ever. There are currently two general trends shaping the evolution of instruments in this field, and they correspond to analytical improvements in the software of existing instruments and to the emergence of a totally new category of instruments — ocular imaging systems that bring the power of multiple imaging capabilities to a single platform.
Today's corneal topographers are smarter than ever. Many systems have a familiar basic configuration that includes some type of reflective Placido target or scanning slit device to image the cornea. Nevertheless, devices today have more sophisticated algorithms to derive corneal shape, detect anomalous features and even design customized contact lenses based on corneal topographic measurements.
The Medmont E300 (Precision Technology Services, Ltd.) is a corneal topography system capable of high-speed capture (30-to-50 Hz). This capability is interesting, not only because it permits rapid acquisition of several images, but it also makes it possible to evaluate the quality and repeatability of images captured. The usefulness of this may not be obvious until you consider the effect that tear quality, eye lids and other artifacts have on corneal imaging. In recent research with the Medmont topographer (Iskander, 2005), tear film artifacts were used to quantify tear film quality. In the search for an objective and quantitative assessment of dry eye (Figure 1), this is an interesting application of corneal topography that could prove useful.
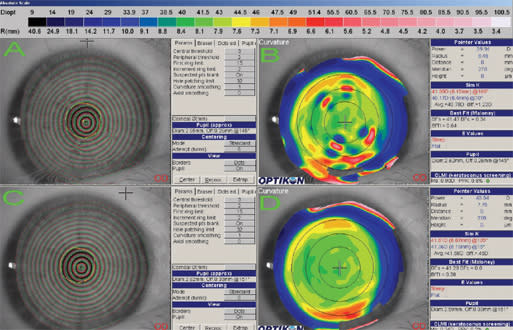
Figure 1. (A) Irregular mires caused by tear film debris that produced the curvature map in (B); (B) irregular corneal topography caused by ocular surface and tear film irregularities seen in (A); (C) the same eye without surface drying and tear film debris; (D) the topographic map associated with the normal ocular surface in (C).
In other research (Alkhaldi, 2003; Alonso-Caneiro, 2008), sophisticated image processing algorithms are used to extract useful images from poor quality data, e.g. reflections from eyelashes or irregularity caused by corneal scarring. While their deployment may not be obvious to casual users, these powerful software routines can help clinicians reliably image a larger area of the cornea. They should also help provide some information in cases in which it was impossible to image before. Look for these and other advances to filter into the marketplace over the next several years.
Interpretation and Display of Topographical Information
Another active area of research is the interpretation and display of topographic information. A persistent problem in refractive surgery and a primary use for corneal topography is the preoperative detection of keratoconus and patients at risk for post-surgical corneal ectasia. Keratoconus detection routines exist on every corneal topography platform, but strategies and performance vary widely. Current strategies are more sophisticated than ever before. Today the problem is not detecting frank keratoconus; the problem is detecting its most subtle variants that one day may become disease. To address this challenge, researchers have deployed machine-learning algorithms that can identify subtle and characteristic signatures of this disease (Twa, 2005). They have even combined multiple features, e.g. corneal thickness, corneal curvature and the location of abnormalities, to build more sensitive detection schemes (Kollbaum, 2008).
The cost of this technological arms race is complexity for users. And while there will never be a perfect way to detect every potential problem case without being overly sensitive, one recently developed index that is available on two commercial systems (Keratron Scout [Eyequip] and Galilei [Ziemer Ophthalmic Systems AG]) is a big improvement over other methods. In their most simple form, keratoconus detectors alert users to a potential problem by identifying irregular or asymmetric contours. This new index, the Cone Location and Magnitude Index (CLMI), provides a statistical probability of abnormality along with a graphical indication of where the problem lies (Figure 2) (Mahmoud, 2008). This approach not only identifies potential problems, but provides an indication of the severity and specific location of possible problems.
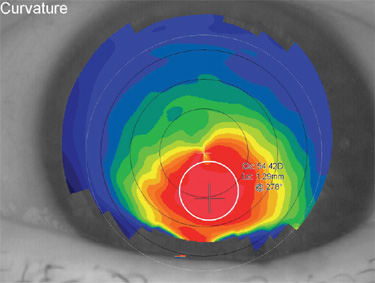
Figure 2. The Cone Location and Magnitude Index is designed to locate the steepest region of the cornea. The routine searches the central 8mm region of the map (the region bounded by the dashed outer white line). Using a smaller circular spot, the algorithm finds the 2mm area with the steepest curvature (solid white line). When the steepest region is identified, the average curvature within the steep zone is compared with a similar zone in the opposite meridian. This helps differentiate astigmatism from asymmetrically steep regions. Then the average curvature inside the steep zone is compared with the area outside the steep zone, and greater differences are associated with higher risk of keratoconus.
This new index will not be the last. Look for future tools to integrate information from multiple risk factors much like cardiovascular risk calculators available today consider a combination of demographic and behavioral characteristics to derive the probability of cardiovascular disease (e.g. http://www.mayoclinic.com/health/heart-disease-risk/HB00047).
Hardware Changes
While analytical improvements have made these devices more powerful and useful, the most fundamental changes have been in the hardware arena. Over the last few years, several new instruments have come on the market based on the scanning slit technology first introduced by Orbscan (Bausch & Lomb). The Pentacam (Oculus Pentacam) was the second scanning slit-based instrument to market and has been a popular item in refractive surgical practices. It has a single axially rotating camera system with several hardware and software improvements intended to increase the resolution and coverage of corneal maps. The software has many familiar mapping options and several new routines such as corneal wavefront calculations intended to help clinicians appreciate the visual consequences of structural abnormalities.
More recently the Galilei corneal topography system was introduced. This instrument is based upon a dual rotating Scheimpflug system that integrates a circular Placido target as well (Figure 3). This dual camera system provides more precise triangulation of surface points — a big advantage over single camera systems — and will have the capability to integrate curvature and elevation information for more accurate descriptions of irregular corneas.
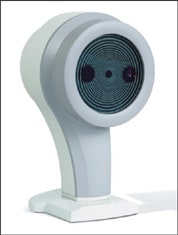
Figure 3. The Galilei is a new topography device based on a dual scanningslit corneal imaging system.
One of the more mature topography platforms, the Keratron corneal topography system (Optikon 2000, Eyequip) has continued to evolve as well. Eyequip's first topography system (Keratron) was selected as the common platform for use by the Collaborative Longitudinal Evaluation of Keratoconus investigators back in the 1990s. The Keratron Scout was the company's next product. This instrument was the first to eliminate the need for another large instrument cart in the examination lane. Eyequip released its newest instrument, the Piccolo, in 2008 and has taken miniaturization and portability to another level (Figure 4). The Piccolo runs on the same mature software platform as the Scout that includes features such as corneal wavefront calculations, pupillometry and CLMI for keratoconus detection. The software also includes one of the most powerful design/simulation packages available for making custom soft, rigid and orthokeratology lenses. The Piccolo can be hand-held or placed in the Hruby lens mount of any slit lamp. This topographer is slimmed down in format and price, but maintains the measurement capability and analytical features of devices with a much larger footprint.
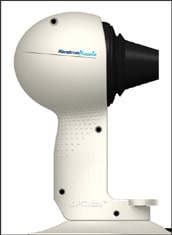
Figure 4. The Piccolo is the newest corneal topography system released by EyeQuip.
The Orbscan has always been more than a corneal topography system and is capable of providing corneal thickness measures, anterior chamber depth, irido-corneal angle estimations and even curvature of the anterior lens surface. The most recent modification to this system has been the creation of a single workstation (Zyoptix, B&L) to house the Orbscan and the Zywave wavefront sensor companion product. This is an incremental step toward another major development in the entire field — the full integration of multiple measurement devices. For now this system requires two instrument heads. Look for new devices to combine these measurement capabilities into a single head.
Nidek achieved this goal several years ago by combining a high-resolution autorefraction system (ARK-10000) with corneal topography to create the OPD Scan. Nidek's software is capable of mapping the corneal surface and describing optical aberrations of the eye in sophisticated ways such as point spread functions, modulation transfer functions and even simulated acuity charts. Likewise, Topcon has combined a Hartmann-Shack wavefront sensor with a corneal mapping system, the KR9000PW, to provide these same capabilities.
The iDesign is a prototype product under development by Advanced Medical Optics that will also fit into this combined platform segment. What is unique about this device is the way in which it derives corneal shape. It does not depend on the traditional Placido target, but instead uses a grid-based pattern of reflective spots to detect corneal shape. In a sense, this is like using the grid pattern of a wavefront sensor on the cornea. By observing the pattern of grid distortion caused by corneal shape, it is possible to determine the corneal contour. Another unique advantage is that this corneal mapping is combined with the highest resolution and dynamic range commercially available for the measurement of ocular wavefront errors, the COAS HD.
A Look at the Future
For some time in the world of refractive surgery, all emphasis has been on the measurement and elimination of optical aberration, and ocular wavefront measurements were the chief concern. The pendulum is finally beginning to swing back toward an appreciation for the interdependence of corneal shape, optical quality and vision. In truth, both matter, and new instruments capable of simultaneous capture of corneal shape and ocular wavefront aberrations are more powerful, cost effective and practical.
Combining these measurement capabilities does pose potential problems. First, as imaging instrumentation becomes more sophisticated, so too must its users. Will these new devices become so complicated that they require a dedicated technician to use them properly? Will correct interpretation of the examination results be a simple exercise that practitioners can include in a busy clinical environment? Second, the evolution of these combined instruments has raised new and important questions about the correct way to use this information.
In a recent publication, Ray Applegate and colleagues (2009) address one of these: the importance of selecting a common and visually relevant point of centration for the measurement of corneal topography and ocular wavefront aberrations. Just as contact lens practitioners know the detrimental visual consequences of a decentered orthokeratology lens, it is imperative that refractive surgical treatments be planned and delivered at the correct location for optimal visual results. These new combined platforms are intended to help achieve these goals.
Another exciting development in the field of corneal imaging is the application of optical coherence tomography (OCT) to the anterior segment. OCT imaging is analogous to B-scan ultrasound imaging. A major advantage of this approach is the ability to resolve structures with near histological detail (Figures 5 and 6). Early uses of this technology focused on posterior segment structures such as the optic nerve and retinal diagnosis. More recently, this technology has been adapted to image the anterior segment. Much like the Orbscan, a sequence of slit images is produced that can be used to construct a detailed description of the entire anterior segment. This application of OCT technology is in its infancy, but holds promise for another evolutionary leap.
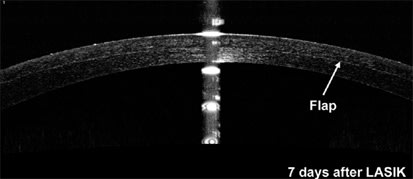
Figure 5. The RTVue is a spectral domain OCT capable of imaging the anterior segment. This device currently provides thickness mapping of the corneal surface along with routines for keratoconus detection. This image was acquired seven days after LASIK. The boundary of the LASIK flap is clearly visible, and software analysis tools can be used to easily measure the thickness of the flap.
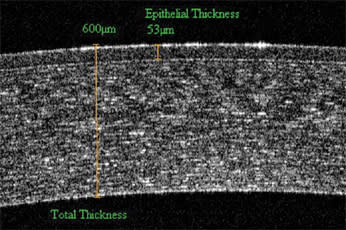
Figure 6. OCT imaging reveals the internal structure of the cornea. The epithelial boundary is clearly visible, permitting users to make measurements of both total corneal thickness as well as epithelial cell thickness.
The first instrument capable of anterior segment imaging was the Visante (Carl Zeiss Meditec). This instrument provides corneal thickness measurements, detailed descriptions of the internal corneal structure and views of the irido-corneal angle, but it was not designed to provide corneal topography. Newer spectral domain OCT instruments (SD-OCT) such as the Optovue RTVue (Optovue), have a resolution of about 5μm and can provide corneal thickness mapping along with keratoconus detection.
What is exciting about this technology is that it can reveal the ultrastructure of the corneal tissue with unprecedented detail — the type of resolution that would normally require sending tissue samples off to a histology laboratory. One can measure corneal and epithelial thickness, view the structural anomalies caused by corneal dystrophies and much more. At present this technology is not capable of providing a good description of corneal topography, but with a better understanding of what fundamental structural changes present in early disease, this type of imaging technology may allow us to detect disease even before corneal topographic changes are evident. The evolution of corneal imaging has come a long way and is far from finished. CLS
To obtain references for this article, please visit http://www.clspectrum.com/references.asp and click on document #159.