CONTACT LENS SURFACES
Developments in Contact Lens Surfaces
Advancements in contact lens surface technology aim at reducing lens-related discomfort and complications.
By Ross Grant, MSc, FCOptom
The arrival of silicone hydrogel lenses has largely addressed the issues of corneal oxygen supply, and the focus of research has moved to other topics—among which surfaces appear prominently. This article reviews the basic model of the ocular surface, the effects of putting a contact lens onto it, and what is being done to make contact lens surfaces best fit into this environment.
The Ocular Surface
The ocular surface can be divided broadly into the corneal and conjunctival surfaces, whose primary functions are refraction and lubrication/protection, respectively. The tears convert the rough, hydrophobic surface of the epithelium into a glassy-smooth, liquid refractive surface. They comprise a base layer of mucins secreted from the epithelia that key into the microvilli of the epithelial cells, which is overlaid by mucus from the goblet cells that is smeared during blinking, which is overlaid in turn by the aqueous tears that contain soluble mucins from the lacrimal gland (Ramamoorthy and Nichols, 2008). The lipid layer, laid down by the meibomian glands, restricts evaporation and maintains the integrity and smoothness of the surface so that it can perform its refractive purpose; breakup of the smooth surface is associated with visual disturbance (Liu, et al, 2010).
The conjunctival epithelium lubricates and protects without the cornea's need for optical clarity. Goblet cells and the epithelia produce mucins to hydrate and lubricate. In the event of infection or irritation, mucus production increases, and strings of mucus collect organisms, cells, and debris, which they wrap up and carry to the lower fornix and then the inner canthus for removal. The tears contain lysozyme, lactoferrin, and other immune system components, and the bulbar and tarsal conjunctiva is richly vascularized, ensuring a rapid and strong response to infection or irritation.
The Effect of Contact Lens Wear
Applying a hydrogel contact lens changes this environment. The lens takes up some of the tear volume, and the pre-lens tear film becomes relatively thinner, averaging about 2.0 to 2.5 microns (Nichols et al, 2005; Nichols and King-Smith, 2006), compared with the pre-corneal tear film thickness of 4 microns (King-Smith, 2000). The tear film over the lens is also relatively less stable (Alonso-Caneiro et al, 2009). The lens is occlusive, covering the cornea, limbus, and a small area of surrounding conjunctiva, whose surfaces are no longer directly swept by the tarsal conjunctiva nor bathed by the renewed aqueous tears during blinking. The mucus on the surfaces under the lens therefore must be replenished, either directly from the underlying secretory surfaces or gradually from the edges of the lens as a result of movement.
Shortly after application, a contact lens is coated in tear components such as mucins, proteins, and lipids, some of which remain on the lens surface while others diffuse into the lens material (Tighe, 2004). These are all naturally occurring parts of the tears, and in coating the lens will help to make it compatible with the surrounding tissues that are accustomed to the presence of the coating components. With time, exposure to air, and/or interaction with the lens surface, the lens deposits can become oxidized (Panaser and Tighe, 2010) or denatured. This can alter their surface characteristics or make them “foreign,” potentially triggering allergic immune responses such as lens-related papillary conjunctivitis. The concept of compatibility, in which the contact lens does not disturb the surrounding environment, is supplemented by that of biomimesis, in which it behaves in the same way as the surrounding tissues to better blend into its environment.
Contact Lens Polymer and Surface Influences
The lens surface acts as the interface between the contact lens and the surrounding tissues, so it is important for good clinical performance. Polymer chemistry allows many surface characteristics to be designed and engineered. Selected characteristics depend very much on the design life and modality (daily wear, extended wear) of the required lens. A one-month replacement, extended wear lens needs to maintain a stable and compatible surface for the full month; deposition with a daily disposable lens is less of a concern compared to the repeated exposure of a new surface to the ocular tissues. A number of measurements can give us information on lens surfaces, and we need to be able to relate these to clinical performance.
Wettability describes how readily a surface will allow a film of water to spread over its surface. It is a reflection of the attraction, or energy state, between the surface, the liquid, and the surrounding air—low attraction/high energy causes the liquid to resist spreading and to stand clear of the surface, forming beads; high attraction/low energy causes it to spread more readily across the surface in a film. At any interface, the materials on either side will seek to create the lowest energy state, which means that hydrophilic and hydrophobic elements will seek out other elements most like themselves—hydrophilic to hydrophilic and hydrophobic to hydrophobic.
Good wettability allows a smooth tear film to spread over the lens, providing a good refractive surface. Poor wettability results in breakup of the pre-lens tear film, with consequences to the clarity of vision (Liu et al, 2010). The relationship between comfort and wetting angle is not yet clear—Cheung et al (2007) found little difference in comfort between etafilcon A lenses, which have a wetting angle of about 22 degrees (Haddad et al, 2010), and galyfilcon A lenses, which have a wetting angle of 109 degrees (Nash et al, 2008), suggesting low correlation. Once lenses are placed on the eye, wetting angles that are significantly different ex-pack resolve to very similar (and low) values within 30 minutes of wear (Haddad et al, 2010), probably because all lenses become coated with tear components. Varying comforts of newly applied lenses gradually converge until there is no difference among them (Dumbleton et al, 2008), suggesting, but not confirming, a correlation between wetting angle and initial comfort.
The molecular structure of silicone (siloxane) has considerable free space, allowing oxygen to diffuse through it quickly (Nicolson and Vogt, 2006). This loose structure also means that it is relatively easy for polymer chains appended to the polymer backbone to rotate around the backbone to a different position (Tighe, 2004). When a lens is immersed in saline in its blister before use, hydrophilic parts of the material position themselves readily at the surface. During wear, the front surface of the lens can dry out, creating a hydrophobic environment. The hydrophilic portions tend to rotate to a position inside the lens, which is still moist, while hydrophobic siloxane portions rotate to the dry environment at the surface, creating the lowest energy solution for both. With repeated exposure, more hydrophobic components move outward, creating patches to which hydrophobic lipids and proteins are attracted. As these accumulate, a cycle of further rotation and deposit attachment extends the dry patches. This breaks up the tear film, which in turn disrupts vision and comfort, rubbing the inside of the lid and its margin during blinking. To counter this, several strategies have been employed to render silicone hydrogel lens surfaces hydrophilic (Winterton and Su, 2004; Nicolson and Vogt, 2006).
Plasma treatments use ionized gas in a vacuum to alter the energy characteristics of lens surfaces. The ionization comes from a high-frequency electrical discharge, and gases can be varied to achieve different effects. Oxygen gas and a relatively low energy level etches the surface of the material by reacting with the outermost molecules, giving yield to hydroxyl moieties such as those found in HEMA. However, the ability of the polymer chains to rotate these away from the surface makes the change too transient; therefore, a higher energy level is used to oxidize the siloxane in the surface to silicates, which are glassy. Such a surface, found on PureVision (Bausch + Lomb) lenses, features glassy islands that are hydrophilic and enable water to bridge the gaps among the islands in which the underlying siloxane is still manifest.
A mixture of trimethylsilane oxygen and methane in the plasma chamber deposits a thin (25nm), smooth, flexible coating of another material that attaches covalently to the surface, rendering it hydrophilic (Teichroeb et al, 2008). This approach has been used by Ciba Vision in its Air Optix, Night & Day, and O2Optix Custom lenses. The Premio (Menicon) lens plasma treatment incorporates both oxidation and the addition of a new material to the surface (Jones, 2007).
In its Acuvue Advance and Acuvue Oasys lenses, Vistakon incorporates a hydrophilic entity, such as high-molecular-weight polyvinyl pyrrolidone (PVP), into the polymer so that it extends beyond the lens surface, shielding the siloxane beneath it. PVP is also used in 1-Day Acuvue Moist (Vistakon) daily disposable lenses, where it is locked into the lens polymer to enhance hydrophilicity. Vifilcon A hydrogel lenses have a slippery surface arising from the slow release of non-functionalized PVP from the lens.
CooperVision, in its Biofinity and Avaira lenses, incorporates hydrophilic “links” into the polymer chain, attached to the siloxane groups to render them more hydrophilic. This obviates the need for additional wetting agents on or in the surface.
Two possible approaches to improve wettability that have not yet been used are surface grafting, in which long hydrophilic molecules are attached to the surface to create a “brush” that sticks out from the surface, and layer by layer, in which alternating an-ionic and cationic layers are added to the surface by soaking in solutions to reach the desired state.
Wettability is quantified by measuring the contact angle (the angle at an interface bounded by the contact lens), the liquid (usually, but not always, purified water), and air. The contact angle is an indication of the level of attraction, or energy, between the materials on either side of the interface. A small angle means that the attraction is high, and the liquid will spread easily as a film with relatively little energy, while a large angle corresponds to a high energy state with little attraction, causing the liquid to stand away from the surface. There are three values of wetting angle: advancing, in which the interface is moving across the surface from wet to dry; receding, in which it is going in the opposite direction; and hysteresis, which is the difference between them. The amount of hysteresis is an indication of the mobility of the hydrophilic and hydrophobic groupings and the ability of the surface to change between hydrophilic or hydrophobic states (Tighe, 2004).
The contact angle has been used to try to predict on-eye wettability and can be measured in vitro using the Sessile drop, captive bubble, or Wilhelmy plate methods. The last of these, in which a sample of material is slowly lowered into and raised out of the liquid, is less frequently used with contact lenses as it is relatively complicated to set up. In the Sessile drop technique, a drop of liquid is lowered onto the lens surface from a pipette nozzle to measure the advancing contact angle (Figure 1). With the captive bubble technique, the lens is submerged in a liquid and a bubble is released from a nozzle beneath it to spread across the surface (receding angle), after which the bubble can then be drawn back into the nozzle to measure the advancing angle (Figure 2).
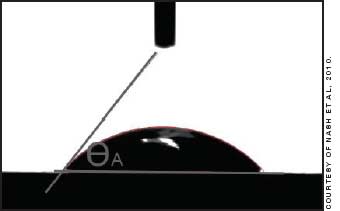
Figure 1. Measurement of advancing contact angle using the Sessile drop technique.
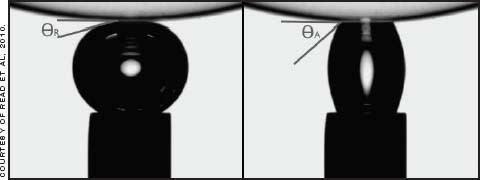
Figure 2. Captive bubble measuring (left) receding and (right) advancing contact angles.
Read et al (2009) found Sessile drop results to be less repeatable, suggesting that this results from the difficulty in standardizing lens blotting before measurement as well as relative humidity and air movement in the air phase of the interface. With the captive bubble the lens does not need to be blotted as it is immersed, and the air in the bubble is not susceptible to surrounding atmospheric conditions. Lenses can be measured ex-pack, to include the effects of any additives added to the packing saline (Nash et al, 2008), or after soaking for some time in saline to exclude these (Read et al, 2009, 2010).
Contact angles measured during (Haddad et al, 2010) or after (Read et al, 2010) lens wear show a reduction in advancing contact angles and hysteresis, suggesting standardization of conditions through coating the surface with tear components. The effect of lipid soaks on contact lens surfaces (Lorentz et al, 2007) demonstrates some wettability improvement for hydrogels, no change or small increases for un-coated silicone hydrogels, and significant improvements for coated lenses.
A more common measurement of surface wettability in the eye is the time taken after a blink for the surface of the tears to destabilize. This can be measured using fluorescein, although introduction of the dye into the tears disturbs their status—and unless a high-molecular-weight fluorescein is used, it can discolor soft lenses. A tearscope (Guillon 1998), enables observation on the eye or contact lens without fluorescein, the result being termed the non-invasive tear breakup time (NIBUT).
Friction and Lubrication Where one surface moves against another with a liquid in between, there will be a gradient of movement in the interstitial liquid. The liquid layer immediately adjacent to the moving surface is dragged by it and then exerts a lesser drag on the next layer, creating a gradient of movement in the liquid (Figure 3). The amount of drag exerted between each surface and the liquid depends on the viscosity of the liquid and the distance between the surfaces. In the eye, this is more complex because of the presence of non-uniform tear components and the extent to which the epithelial surface keys to the mucin glycocalyx (Cher, 2008).
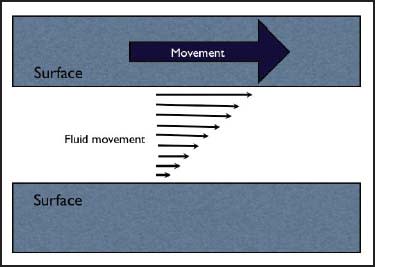
Figure 3. Gradient of movement in a liquid between two surfaces, one moving.
Measuring the force required to move one biological surface over another in standard conditions is called biotribology, and the measurement yielded is the coefficient of friction—the ratio between the tangential force creating the movement and the vertical force between the two surfaces. There are two coefficients: the higher, static coefficient measures the force to initiate surface movement, and the lower, kinetic coefficient measures the force to maintain a steady state of movement (Figure 4).
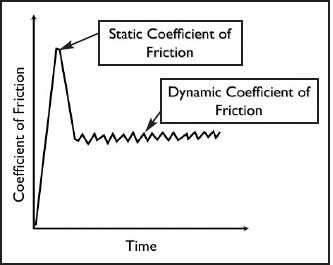
Figure 4. Graphic illustration of static coefficient of friction, occurring at initiation of movement between the surfaces, and dynamic coefficient of friction, which occurs when movement is maintained.
Many wetting agents also have lubricating properties. For example, the PVP used to wet the senofilcon A (Acuvue Oasys) material may also help to give it a low out-of-pack coefficient of friction, which is unchanged by a two-week soak in an artificial tear solution (Moody et al, 2010). In contrast, lotrafilcon B (Air Optix Aqua) and to a lesser extent comfilcon A (Biofinity) lenses show higher out-of-pack coefficients of friction, which improve after the tear solution soak. The recent addition of copolymer 845, a wetting agent, to the blister packs of lotrafilcon B lenses reduces the coefficient of friction by 20 percent (Ross et al, 2010) and could be expected to impact initial comfort until the lens is coated with tear components.
Because of their short life, daily disposable contact lenses can act as a reservoir for wetting and lubricating agents, which can either be locked into the matrix, as with PVP in 1-Day Acuvue Moist, or can slowly elute from the lens to smear across the surface, as with polyvinyl alcohol (PVA) in Focus Dailies (Ciba) lenses. This slow secretion of lubricant, which is spread during blinking and replenished during the day, mimics that of the ocular mucous membranes. Ross et al (2010) report a 55 percent reduction in friction coefficient with 1-Day Acuvue Moist compared with the non-moisturized product. Focus Dailies coefficient of friction measurements with saline soaks between readings are and remain low for four hours compared with three other lenses (1-Day Acuvue Moist, SofLens Daily Disposable [B+L], and Proclear [CooperVision]), in which the coefficient either increases by four hours or starts and remains high (Pruitt et al, 2010).
The latest generation of Dailies (Dailies Aqua Comfort Plus, Ciba) adds two more ingredients to the blister solution to control wettability and lubrication in different parts of the day. The large molecular size of hydroxypropyl methyl cellulose (HPMC) coats the lens surface for initial comfort, and the small molecular size of polyethylene glycol (PEG) diffuses into the lens in the pack, then diffuses out again during the early part of wear.
Roughness An atomic force microscope enables quantification of surface roughness, defined as the average, root mean square, or maximum vertical distance from the ideal position of the surface. Gonzàlez-Méijome et al (2005) showed how the surfaces of three silicone hydrogel lens designs appear relatively smooth at a lower magnification and visibly rougher as the magnification increases. Teichroeb et al (2008) took this further, showing a range of roughness and topographies for hydrogel and silicone hydrogel surfaces that varies by a factor of about three, with omafilcon (Proclear) the smoothest and balafilcon A (PureVision) the roughest.
It is not immediately apparent what this means in the ocular environment. The epithelium itself is not smooth, having microplicae on its surface to which it is postulated that the mucin glycocalyx keys (Cher, 2008). This raises the question of whether there is an ideal roughness to achieve the best mucin coverage on the lens—and whether this is different for different materials.
Antimicrobial Surfaces
The conjunctival sac is a hostile environment for microbes, with its mucus and epithelial surfaces constantly being replaced, the presence of lysozyme and other antibacterials, and the constant wash-through of the tears, churned by blinking. There appears to be no permanently resident population of microbes in the eye as there is in the stomach or mouth. Swabbing yields small, changing populations of organisms (Grant, 1988), which suggests that they are transient, dropping onto the cornea and conjunctiva from the lids and almost immediately being washed away.
Nevertheless, there is an increased risk of infection or inflammatory responses associated with contact lens wear (Dart et al, 1991; Schein et al, 1989; Schein and Poggio, 1990).
The classic infective process is for microbes to attach to a surface and to accumulate into clumps—something associated with favorable conditions for bacteria, whereas in unfavorable conditions they tend to separate. (Sharma 2008). Once established, they communicate with each other in a process called quorum sensing and cover themselves in a slime or glycocalyx that can protect them from antimicrobial agents in the surrounding fluids. When a colony has been formed, the organisms can then invade the underlying tissue.
Contact lens peripheral ulcer and contact lens-associated red eye are not infections, but inflammatory events that appear to be associated with bacteria that are adherent to—or trapped under—the lens and that release toxins, triggering the migration of white cells and other immune components to the site. Contact lenses, therefore, provide a potential vector for bringing microbes into the eye and, by adhesion to their surfaces or occlusion beneath them, providing a foothold for them to cause complications.
One potential way to address this is to create contact lens surfaces that do not allow the inflammatory or infective processes to occur. A number of different approaches are under consideration (Sharma, 2008; Gabriel and Weisbarth, 2009; Weisbarth et al, 2007; McDougall, 2008; Wilcox, 2008). Following are some possible approaches:
• Selenium has achieved 3 log reductions in populations of Staphylococcus aureus and Pseudomonas aeruginosa. It has been successfully used in rabbit trials and short-term clinical wear (Ozkan et al, 2009).
• Silver in the form of nanoparticles in or on the lens polymer releases silver ions in an aqueous environment that kill bacteria by disrupting cell membranes, interfering with DNA and RNA replication, altering respiration, and altering enzyme conformation. Silver has been successfully used to create antimicrobial lens cases, reducing the chances of carry over contamination from the case into the eye.
• Polymeric pyridinium compounds can be covalently bonded to lens surfaces. They kill bacteria by penetrating their walls with polycationic chains.
• Polyquaternium compounds are already used in contact lens disinfectants. They act by chelating metals that are essential for microbial metabolism.
• Lactoferrin is a naturally occurring antimicrobial that acts by competitively binding iron needed by microbes for multiplication, motility, and attachment.
• Defensins are naturally present in neutrophils and macrophages. They bind to the microbial membranes, increasing permeability and lowering their resistance.
• Cationic peptides first penetrate and disrupt bacterial membranes, then the cell contents.
• Lactams and furanones both interfere with quorum sensing as well as adhesion and aggregation of bacteria. Furanones are naturally present on red algae, reducing the attachment of bacteria.
Antimicrobial contact lens surfaces, as with all antimicrobial agents, will need to address the balance of efficacy and nontoxicity before they can come into general circulation. It is not known what effect they will have on infection and inflammation rates with contact lens wear, as these are low and, therefore, not easy to test. Antimicrobial lens surfaces do, however, represent a very exciting area of research, and considerable resources are being allocated to them.
Conclusion
Because of the multiple interfaces and influences that contact lenses have on the eye, their surfaces promise to provide considerable room to improve lens comfort, biocompatibility, and safety. Much still needs to be learned about what makes a perfect contact lens surface—but much is already being done. CLS
For references, please visit www.clspectrum.com/references.asp and click on document #181.
![]() | Ross Grant is a UK-trained optometrist who has worked for over 30 years in the contact lens field, in research, professional services, marketing, and general business management, including 25 years in industry. He is a founding partner in ToolBox Training and Consultancy GmbH, based in Zurich, Switzerland. |