TECHNOLOGY IN CL PRACTICE
New Technology in Contact Lens Practice
Advances in technology are changing the dynamics of contact lens practice and how we fit contact lenses.
By Eef van der Worp, BOptom, PhD, FAAO, FIACLE, FBCLA
![]() |
Dr. van der Worp received his optometry degree from the Hogeschool van Utrecht in the Netherlands and has since worked in different positions at the school. He is affiliated with the University of Maastricht in the Netherlands where he received his PhD degree, is a visiting scientist at Manchester University (Manchester UK) and an adjunct research scientist at Pacific University College of Optometry. He lectures extensively worldwide and he resides both in Amsterdam – the Netherlands and in Washington, DC. |
The dynamics of contact lens practice are changing and will continue to change dramatically in coming years. New technology is available to help us better respect the shape of the cornea, and new instrumentation can help us provide our patients with the best optical correction possible.
Corneal topographers provide us with a great deal of information about corneal shape. Studies have shown that when lenses are fitted more in alignment with the corneal shape, lens comfort is better, corneal physiology is improved, and patients are more satisfied. In addition, anterior segment optical coherence tomography (OCT) can be used for screening and postlens tear film profile imaging, and it allows for better assessment of corneal-scleral shape with potential clinical benefits for soft and scleral lens fitting.
Wavefront technology to measure higher-order aberrations (HOAs) of the eye is becoming a more essential part of our practices, with the potential to eventually replace auto-refraction and even subjective refraction. It can serve to better predict suboptimal visual outcomes with contact lenses, and it can be used for early detection of ocular deviations such as keratoconus. It can also help when choosing whether to prescribe contact lenses or glasses as well as what type of contact lenses. Also, wavefront technology allows improvements to be made to certain types of lenses to optimize vision. In addition, peripheral refraction can become a common procedure on our patients, with the potential for special contact lens applications.
Corneal Topography
Contact lens practitioners have great interest in the shape of the cornea. When this shape is known, a contact lens that will optimize the lens-to-cornea relationship can be selected, designed, and fitted. Generally, mimicking the shape of the cornea promotes lens wearing comfort and reduces mechanical effects of the lens on the cornea.
The standard procedure in contact lens practice is to measure the cornea with a keratometer. But what exactly does keratometry tell us? It typically measures the average curve of the central 3.00mm of the cornea in two meridians. This method has at least three limitations. First of all, a keratometer measures curves, and curves are not the equivalent of shape. Second, it estimates rather than measures the average central curves. It does not provide information about the exact central point (not to mention the apex) of the cornea. Third—and most importantly—3.00mm is a very small area of a cornea, given that a typically cornea is 11.80mm in diameter. Contact lenses cover a much larger part of the cornea than 3.00mm, and they rest beyond this central area.
Interestingly, the principle of corneal topography (the Placido disk) is as old as that of keratometry, dating back to the late 19th century. Corneal topographers can instantly give us information about thousands of data points on the cornea and will provide a better understanding of corneal shape. Practitioners are increasingly relying on corneal shape rather than on corneal curves. Corneal shape is crucial when dealing with refractive surgery, orthokeratology, and keratoconus—but information about the shape of the cornea is also essential for designing and manufacturing any type of contact lens (including soft lenses).
Reflection Topography The most commonly used topography system in contact lens practice is the reflection (Placido disk) system. One problem in interpreting topographic maps of this type is that from them you could determine, for instance, that the radius of curvature somewhere on the cornea is 7.50mm (or 45.00D with a dioptric map)—but what does this mean? Is the cornea steep, or is it a local steepness, or maybe a flatter area on an even steeper cornea? Even more, what is the position of this steeper or flatter area on the slope of the cornea?
It is like walking in an area with hills. You see the curve and you might feel the slope while walking, but are you on a mountain at 10,000 feet or on a dune next to the beach (e.g. at sea level)? To have access to that knowledge, you need a height or elevation map to really know where you are. The same is true with local corneal curvature data. The problem is that a Placido disk inherently estimates radii of curvature whereas a height map would be more ideal. Therefore, most modern corneal topographers also feature “height maps.” Although these maps are still derived from curvature, fast and smart algorithms combined with logical interpolation/extrapolation processes make them reasonably accurate. This data is essential when designing and manufacturing (custom made) contact lenses.
Reading height maps is quite different from reading curvature maps. A height map representing the total, absolute sagittal height is of interest when fitting a soft or a scleral lens, but it would not provide much detail at all. All it would show is that the central cornea is higher than the periphery, which is not really a surprise. It gives you a better idea of the actual shape of the cornea only after matching the corneal surface with a “best fit sphere” (or sometimes “a best fit oval”). Everything that is higher (closer to the observer) than that shape is color-coded with warmer colors; everything that is positioned further away from that shape is presented in cooler colors. This way you can immediately see the exact (and actual) shape of the cornea.
Another problem with reflection systems is that the area that can be measured is still limited because of reflections from the nose and eyebrow, although the area is much larger than with keratometry, of course. Newer topography systems feature smaller cone heads to more easily fit into the orbital opening and result in less peripheral dropout due to reflection. New systems involving color topography and dynamic (live) corneal topography have been developed to better deal with limitations such as peripheral dropout and tear film irregularities.
Projection Topography The disadvantages of reflection systems spurred development of alternatives to the Placido disk-based corneal topographers. Most of these alternative devices are somewhat more complicated to use and more expensive or are used more in research or ophthalmic settings than in routine contact lens practice settings. But they are typically better at measuring a large surface area, and they can measure corneal shape (and sagittal height) directly.
You can easily appreciate the principle of scanning slits that are used in the Orbscan (Bausch & Lomb), Pentacam (Oculus), and Galilei (Ziemer) devices from a routine, cross-section view of the cornea through a slit lamp. In the slit image, the profile of the cornea's front and back surface is visible. Unfortunately this is only one meridian, and by changing the incidence angle of your slit you would see a dramatic change. A scanning slit can obtain multiple images over many meridians. To overcome distortion by different incidence angles, a Scheimpflug correction system is built-in, in some cases with more than one Scheimpflug camera.
From the multiple images obtained, the topographer can compute the corneal shape on both the front and back surfaces. Results are presented in color coded maps that include corneal thickness data. These devices can present height data on both the front and back surface of the cornea with the potential for detecting corneal ectasia in very early stages of the condition. These devices can also perform indirect pachymetry, although the current resolution of such systems is still highly variable and it is not a replacement for traditional pachymetry.
Using Topography Data for Lens Fitting
One of the first things that is obvious when looking at an average corneal topography map is the cooler colors in the periphery of the corneal map, representing relative flattening of the curves. The amount of flattening is traditionally designated as eccentricity, mostly noted as the e-value. The e-value of an ellipse can be calculated from the central curvature and the peripheral curvature plus the distance (angle) from the center where that peripheral curve was measured. The average cornea has been described by many, and its e-value is thought to be around 0.43. But e-value varies widely among individuals, and ideally you should measure and evaluate it for every single eye before fitting contact lenses. As an example, researchers at The Ohio State University evaluated the corneal shape of 683 children's eyes (aged 8 to 15); the vast majority flattened towards the periphery, but two corneas actually steepened.
However, these are average e-values of a cornea, and not all meridians of a cornea have the same e-value. Most corneal topographers and some automated keratometers will provide e-values per meridian or quadrant. A major drawback with regard to e-value measurement is that topographer manufacturers are very secretive about the way they calculate the e-value: neither the distance from the center nor the meridian used are usually revealed, which means that differences in e-values among topographers may occur. Unfortunately, there is no standard for defining corneal shape.
E-value can only describe prolate shapes, whereas corneas that are naturally steeper in the periphery or that are reshaped that way by orthokeratology or laser surgery are oblate shapes. In such cases the e-value is useless, because mathematically it can only define shapes larger than zero.
Furthermore, the actual corneal shape is not as easily defined as a standard ellipse or e-value. Especially towards the periphery, it becomes more complex and less predictable. Corneal shape is usually more spherical near the apex, and it may flatten at a variable (usually progressive) rate towards the periphery. Therefore, newer mathematical definitions of corneal shape have been developed. However, the clinical usefulness of these complex formulations when manually fitting GP lenses seems limited. Therefore, to describe corneal shape, the e-value is still the parameter most used in contact lens practice, and most topographers and automated keratometers currently use this value. An alternative is p-value (mostly used in Great-Britain), which can be derived directly from the e-value.
To obtain a quick (but approximate) idea about the flattening of a cornea (in mm), square the e-value provided by the topographer or automated keratometer. An e-value of 0.4 means that the flattening in the periphery at 30 degrees from the center is about 0.16mm. An e-value of 0.6 describes a flattening of approximately 0.36mm, and a cornea with an e-value of 0.8 is about 0.64mm flatter in the periphery. From this it can be concluded that as the e-value goes up, flattening increases progressively. This also means that small e-values are clinically of little importance and that the importance of e-values increases rapidly as they get higher. The e-value of a keratoconic eye can easily exceed 1.4, which translates to something in the order of 2.00mm of flattening and is highly clinically relevant.
When fitting toric corneas with GP lenses, use the e-value to evenly distribute lens pressure over the corneal surface as much as possible, as you would with spherical lenses. Non-toric lenses on with-the-rule corneas will create pressure in the horizontal meridian. The majority of corneal astigmatism is with-the-rule, as both oblique and against-the-rule astigmatism are fairly rare. Textbooks generally advise using back toric designs when the central corneal toricity is 2.50D to 3.00D or more (based on keratometric data). However, using non-toric lens designs on toric corneas below these values could easily result in topographical changes and spectacle blur.
When fitting a back toric GP lens, it's usually recommended to fit the flattest meridian in alignment with the cornea, taking radius of curvature and the evalue into account. The other meridian is generally fitted slightly flatter than alignment to create a lens that moves well, but also to compensate for the difference in refractive index between the tears and the lens material and thus to prevent induced astigmatism. A standard popular rule of thumb used frequently in the past to compensate for astigmatism induced by the tear layer was to take two-thirds of the corneal astigmatism and add this to the flattest meridian to calculate the steepest meridian. However, with the highly sophisticated lathing technology currently available, it is technically easy to compensate for the induced astigmatism on the front surface of the lens. Hence, don't worry about induced astigmatism when fitting toric GP lenses, focus only on the lens fit itself.
Still, a slightly flatter-than-alignment base curve radius (BCR) in the steepest meridian is desirable to promote movement, but should be no more than 0.75D.
If lower degrees of corneal toricity are present (in particular, when peripheral corneal toricity is apparent), toric back-surface geometries with one spherical and one aspheric meridian can be used. Usually one meridian is fitted with a low or zero e-value, while the flattest meridian is fitted with an e-value between 0.6 and 0.8. It is advised to use large diameters because the toricity increases towards the periphery. Be aware that, when evaluated with a radiuscope, these lenses are spherical centrally and start to diverge toward the lens periphery (and they will not show toricity on the radiuscope). The contact lenses are marked in the flattest meridian to help in evaluating the lens position on the eye; they should demonstrate no or limited signs of rotation during lens wear.
In addition, corneal toricity may increase or decrease towards the periphery and thus influence fitting characteristics. Do not neglect this effect of peripheral astigmatism when fitting GP lenses, because these lenses rest mostly peripherally. Central corneal astigmatism is easier to manage compared to limbus-to-limbus corneal astigmatism when fitting GPs. Corneal topographers can help you assess the degree of peripheral astigmatism. If a topographer is not available, the fluorescein pattern of a standard non-toric trial lens placed on the eye will help you assess how much corneal toricity is present and whether this is acceptable or not. Also, quadrant-specific lenses are now available in both soft and GP designs.
Corneal shape data can help with soft lens fitting as well. However, we have diverted so much from actually fitting soft lenses that most practitioners don't understand the soft lens designs that they fit on a daily basis. For example, most daily disposable contact lenses are monocurves, but it is clear that the cornea cannot be defined as a single curve. And changing the BCR of a soft lens very often does not create the desired effect (e.g. more or less movement). Why is this so? Again, it is related to the peripheral curves of the cornea and even to the anterior scleral shape. We should therefore fit soft lenses based on sagittal height information (e.g. elevation data) rather than on central curves.
Furthermore, Szczotka et al (2002) found that peripheral corneal toricity was one of the major factors determining the success of toric soft contact lens fitting and ideally should be considered. Success with fitting soft toric lenses may increase using this information. Also, you can optimize the success of soft multifocal contact lens fitting by taking the corneal topographical data (locating the actual top of the cornea) into account.
Improving Corneal Physiology Corneal warpage is not uncommon in daily contact lens practice, although its magnitude can differ dramatically. It is wrong to assume that it occurs only with GP lenses: it is estimated that at least one-third of corneal warpage cases come from soft lens wear (Figure 1), so take caution when evaluating soft lens wearers who are candidates for refractive surgery.
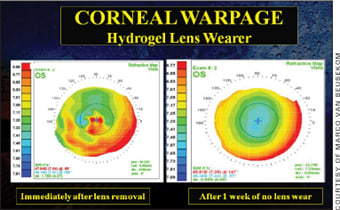
Figure 1. Corneal warpage resulting from soft lens wear.
Silicone hydrogel lens materials were thought to dramatically decrease corneal warpage by reducing hypoxia. But changes to the corneal surface still occur with silicone hydrogel wear, most probably because of the increased modulus of some materials. Recent research even indicates that lens care regimen (peroxide versus multipurpose solution) may have an effect on lens fit and consequently can lead to unwanted corneal changes and corneal warpage. In addition, there seems to be increasing evidence that postlens tear film exchange is of utmost importance in optimizing corneal physiology and decreasing the chance of developing corneal infiltrates.
For GP lenses it has been demonstrated that using corneal topography can significantly improve the lens fit. Apart from preventing unwanted corneal changes, it is also helpful in managing 3 o'clock and 9 o'clock staining and therefore is beneficial to corneal physiology. Conversely, controlled and desired corneal changes such as in corneal reshaping (orthokeratology) can be monitored, controlled, and perfected by using this device.
Optimizing Lens Comfort Studies at the University of Maastricht in the Netherlands show that if you compare GP lens comfort between a group of lens wearers who have “optimal” lens fits versus a group who have “suboptimal” lens fits, there is a 2-point difference on a 10-point scale in comfort between these two groups at three months, which is a statistically and clinically significant difference. This implies that even small improvements in GP lens fits could influence their comfort during wear. You can improve GP lens fits by using corneal topography.
The other interesting result from this study is that some corneas with lesser degrees of central corneal astigmatism can still exhibit toric fluorescein patterns because of an increase in corneal astigmatism towards the periphery. Patients who have such corneas would therefore benefit from modern back toric lens designs, which would provide improved comfort compared to non-toric lens designs.
In soft lens wear a suboptimal lens fit can also lead to reduced lens wearing time and to variation in visual acuity. If a soft lens fit is too steep for the cornea, some central corneal clearance occurs underneath the lens. Vision is good immediately following the blink (when the clearance is still minimal), but shortly thereafter vision and potentially comfort is reduced when the lens takes its original shape and the clearance reappears. Possibly, these lens fitting characteristics are more critical with silicone hydrogel lenses than they are with traditional soft lenses. These phenomena are currently under investigation at the University of Maastricht.
Anterior Segment OCT
Recently, devices have been developed that are able to image the front segment of the eye based on OCT. The principle behind these devices is a Michelson interferometer in which time differences using two imaging paths from the same target are used to compute distance data. One imaging path is calibrated for the device; the other path contains the object to measure, in our case the eye. This allows the anterior segment and the cornea to be measured using high resolution imaging. From these images the real sagittal height of the cornea can be obtained. This information can help improve soft lens fitting and help with designing better soft contact lenses for the future, as discussed in the previous section.
OCT can also be used to map the anterior sclera. Large-diameter contact lenses that have their resting point beyond the corneal borders (scleral and semiscleral contact lenses) are believed to be among the best vision correction options for irregular corneas; they can postpone or even prevent surgical intervention as well as decrease the risk of corneal scarring. But not much is known about the shape of the corneal-scleral transition area. Studies with OCT have revealed that on average, the limbus on the temporal side of the cornea appears to be less pronounced than it is on the nasal side. Basically this means that the nasal scleral portion appears “flatter.” This seems to be in line with corneal topography findings: the flattening that occurs in the nasal portion of corneal topography maps seems to extend out into the sclera (Figure 2).
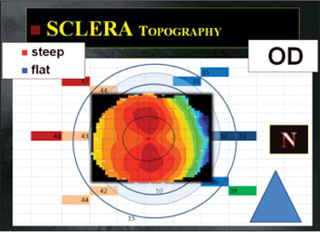
Figure 2. Mapping the entire anterior segment surface of the eye: Example of anterior scleral angles in the right eye of a subject in relation to corneal topography of the same eye (Pacific University).
The shape of the limbal area and the first part of the sclera beyond the limbal area is a subject of discussion. Previously it has often been assumed that the sclera has a curved shape, but this is not always the case. From the molds taken of the anterior segment of human eyes (in normal and in keratoconic eyes) and from OCT, it appears that from the peripheral cornea outward, the sclera often continues in a straight line (e.g. is tangential, Figure 3). Also, when using contour maps from the Maastricht Shape topographer—one of the first topographers to image up to an 18mm diameter of the anterior eye surface including the limbus and part of the sclera—it seems that the transition is often tangential rather than curved. From pilot studies independently performed at Pacific University and the University of Waterloo (Canada), it appears that the peripheral angle is roughly 40 degrees on average (unpublished data).
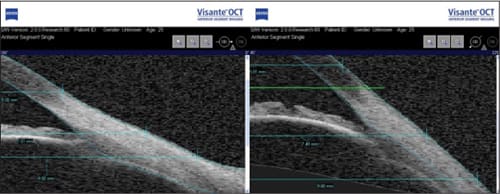
Figure 3. Flat (left) versus steep anterior scleral shape as shown with the Visante (Carl Zeiss Meditec) anterior segment OCT (Pacific University).
Based on clinical input it appears that one or more sections of the sclera are often steeper or flatter compared to the rest. The non-rotationally symmetric nature of the sclera has been described previously by Visser et al (2006) in a paper on toric scleral lens designs. In fact, toric designs are used today more often than not when fitting scleral lenses in Visser's practice in the Netherlands—one of the largest scleral lens practices in the world. But from studies at Pacific University it appears now that not only can the sclera be toric, but truly non-rotationally symmetric in nature in which one segment is steeper or flatter than the rest. This would require a quadrant-specific scleral contact lens, which is available now in the United States.
Wavefront Aberrometry
It is estimated that correcting sphere and cylinder (the second-order aberrations) refractive errors eliminates approximately 80 percent to 85 percent of all aberrations of the eye in an average individual—but a substantial amount of the remaining 15 to 20 percent is not corrected. Although there are dozens (hundreds in fact) of HOAs, the third- to the sixth-order HOAs are the most important in terms of clinical relevance.
It appears very simple: measure all of the optical aberrations of the eye and by some means provide a perfect correction. We could produce a spectacle lens incorporating a correction for all aberrations of the eye. It would optically be a very strange lens but it would enhance the retinal image. However, the patient's eye would have to remain very static in relation to the corrective lens. Any movement of the eye behind the spectacle lens would immediately degrade the perfect image. Because the spectacle lens is placed at some distance from the eye (vertex), the effects on induced aberrations by a change in fixation are dramatic.
GP Lenses Spherical GP lenses can correct HOAs much better because the lens centers at the cornea and moves with the eye to a large extent, but excessive lens movement and decentration can still be a limiting factor. If we apply a toric GP lens to the same eye, the situation improves significantly because stability is improved. The tear film acts as a “wavefront aberration lens,” correcting most of the anterior surface aberrations (90 percent [Figure 4]—the rest are lost due to the difference in index of refraction between the cornea and the tear film), but it does not correct all internal aberrations. This is comparable to correcting astigmatism: usually a GP lens is better, but sometimes the internal astigmatism is “activated” by correcting the anterior surface optically, with a resulting negative effect. In those cases, although rare, a soft lens would be preferred. But generally the quality of the retinal image is substantially improved with GP contact lenses, which also brings the contrast sensitivity function up to the highest level, thus serving as a natural wavefront-correcting lens.
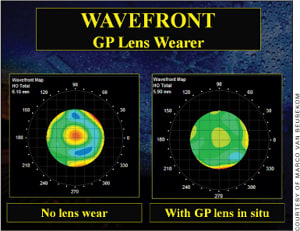
Figure 4. Wavefront aberration maps with and without a GP lens in situ showing the total higher-order aberrations, taken with the iTrace (Tracey).
Wavefront aberrometry can also detect corneal irregularities in very early stages—even before any clinical signs are present. For instance, research shows that HOAs—primarily coma—are more prominent on both the anterior and the posterior poles of the cornea in keratoconic eyes compared to normal eyes. Interestingly, it seems that residual irregular astigmatism in keratoconus patients who wear GP lenses can be estimated by measuring the HOAs from the posterior corneal surface alone.
Soft Lenses It is evident that HOAs will play a more important role in the future of soft lenses. For instance, in symptomatic wearers of disposable soft contact lenses, the total HOAs when wearing lenses with polyvinyl pyrrolidone (PVP) are significantly lower than with traditional lenses. It seems that sequential measurement of HOAs may be a useful objective method to evaluate the effect of internal lubricating agents in disposable soft contact lenses on optical quality and on ocular dryness in general.
The image quality of standard soft lenses can be further improved by using wavefront aberrometry as well. There are two ways of achieving this: by using rotationally symmetrical lenses, or by manufacturing non-rotationally symmetrical lenses. For the first category this primarily means manufacturing lenses that better correct the HOAs of the average eye. Spherical aberration, which is present in most normal eyes, can be easily corrected, and many manufacturers are incorporating this into their soft lenses. This is good news for patients who have average eyes, but for patients who have not-so-average eyes such lenses may work contrary for them. Perhaps different aberration profiles (with low, medium, and high spherical aberration correction, for instance) will be available to future contact lens fitters to optimize optical quality in most soft lens wearers.
The alternative is to create a custom contact lens for each individual eye. At the University of Maastricht we have measured the wavefront aberrations in an average, well-centered contact lens. Contact lenses must move with the blink and they also rotate on the eye, and this translation and rotation degrades an ideal correction. We have found that in severely aberrated eyes such in keratoconus, translations of more than 0.50mm can degrade the retinal image such that the gain in visual function is lost and the image quality actually gets worse. Rotation is less critical: 10 degrees of rotation can be acceptable. This means that for a complete correction of higherorder optical aberrations, the contact lens must be designed such that it achieves optimal stabilization on the eye. This requires a fully customized contact lens. Currently, a few different wavefront-corrected soft lenses are available that are manufactured with lathe-cut techniques—some are even offered in silicone hydrogel materials.
What the Future Has in Store
This brings us back to the technology in contact lens practice that was mentioned earlier: corneal topography. Corneal topography can help us create custom-made back surfaces for soft and GP lenses that mimic the cornea and result in better centered and more stable lenses. Scleral and hybrid lenses especially have a great potential in this regard—they have the ability to create stable, optimized optical systems, especially in eyes that such lenses are particularly indicated for (e.g. keratoconus, etc).
In addition, by looking at aberration profiles of individual eyes, we should be able to create better custom multifocal contact lenses for our patients in the near future. We also may be able to induce controlled changes of the corneal surface with corneal reshaping techniques that can help optimize the optical outcome. One suggested optimal outcome is to create a pattern that can correct the relative hyperopia present in the periphery of myopic eyes. This appears to have potential applications for slowing myopia progression in children. More recent work has demonstrated that multifocal soft lenses create the same optical pattern (with the lens in place) on the cornea, possibly also helping to reduce myopia progression in children.
We have only begun to understand the potential for how new technology can improve the quality of contact lenses and contact lens fitting. This article provides only a glimpse of what impact new technology potentially can have on the contact lens practice of the future—in which there someday may not be a place for manual subjective (central) refraction and trial lens fitting. CLS
To obtain references for this article, please visit http://www.clspectrum.com/references.asp and click on document #171.