Underwater Vision
Improving Underwater Vision: Part 2
The authors explore the potential of a lens designed to provide clear vision in air and in water.
Dr. Schwiegerling is a professor of Optical Sciences and Ophthalmology & Vision Sciences at the University of Arizona. His research interests include contact and intraocular lens design as well as development of ophthalmic instrumentation. He is a consultant or advisor to Visioneering and has received research funding from Alcon and Wavetec. |
Dr. Legerton is a vision scientist, author, and inventor with 34 U.S. patents for contact lenses, refractive surgery, and ophthalmic instruments. He is a stock shareholder of Innovega Inc., Vicoh, LLC, Preventive Ophthalmics Inc., Myolite, Inc.; an employee of Innovega Inc.; and a consultant to Paragon Vision Sciences and Carl Zeiss Vision. |
Dr. Chou is a partner at EyeLux Optometry in San Diego and an author for eyecare industry publications. He enjoys snorkeling. He is a consultant or advisor to Transitions Optical and has received research funding from Alcon and Paragon Vision Sciences. |
Dr. Joyce is in private practice in Southern California and is a clinical investigator for the contact lens industry. He enjoys scuba diving and surfing. |
Dr. Meyers is the vice president of Science & Technology for Paragon Vision Sciences. He is an inventor on more than 30 patents for biomaterials and contact lens design including those for Paragon CRT and the NormalEyes 15.5 mini-scleral lens. |
By Jim Schwiegerling, PhD; Jerome A. Legerton, OD, MS, MBA, FAAO; Brian Chou, OD, FAAO; Robert J. Joyce, OD, & William Meyers, PhD
One of the interesting adaptations in nature is found in the so-called “four-eyed” fish Anableps anableps (Figure 1). These natives of Central and South America spend most of their time at the surface of water with the water meniscus bisecting their eyes. Despite their nickname, they have just two eyes. The dorsal half of each eye is in air, while the ventral half is submerged. Each eye has two pupils, divided by a band of tissue and connected by a portion of iris. Each crystalline lens is asymmetric, and one area of retina is adapted to vision in air while the other is adapted to vision in water (Figure 2).
Few humans would choose to have eyes like the four-eyed fish just to achieve amphibious vision. So what can be done for people who would benefit from such vision?
Early Inventions
For water enthusiasts, masks and goggles can afford clear vision, but they are bulky and offer a limited field of view. In 1963, Sports Illustrated published an article about a novel scleral contact lens for underwater vision (Phinizy, 1963). The lens had an air interface to behave like a tiny face mask. The concept never caught on, and nearly 50 years later, the primary method for underwater vision for divers and swimmers is still a traditional mask or goggles. Having unimpeded underwater vision is still as attractive today as ever, yet there have been few technological advances in this realm and no resulting efforts to commercialize a viable solution.
In 2007, we described the optical limitations that challenge underwater vision along with several strategies to surmount them (Chou et al, 2007). At the time, we were working with a hybrid contact lens design with a high-powered center add to allow amphibious vision. Since then, we have shifted to a mini-scleral design in a GP material. In this article, we describe our latest work.
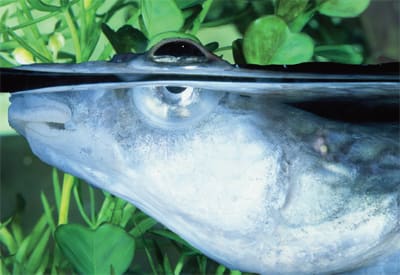
Figure 1. Anableps anableps (four-eyed fish).
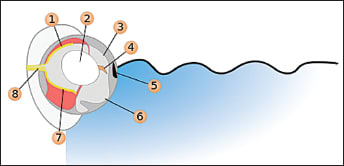
Figure 2. A. anableps optics: 1) underwater retina; 2) lens; 3) air pupil; 4) tissue band; 5) iris; 6) underwater pupil; 7) air retina; 8) optic nerve.
Latest Contact Lens Design for Amphibious Vision
We chose a mini-scleral design in a GP material for several reasons. First, there is nearly universal concern that wearing contact lenses in water increases the risk of microbial keratitis (Kilvington et al, 2007; Boost et al, 2008; Lindsay et al, 2007). GP materials come in higher Dk values compared to the hybrid design we had been working with and offer potentially stronger antimicrobial activity because of the higher decimal reduction values of GP care products when compared with hydrogel care products (Chou, 2006; Rosenthal et al, 2002). In addition, mini-scleral designs have better stability on the eye during impact and in water.
We selected the NormalEyes 15.5 mini-scleral design for our amphibious lens (Legerton, 2010) (Figure 3). Because of the small index difference between water and the eye, the power in the corneal plane must be 135D, on average, to be equivalent to the average of 43.50D in air. The radius of curvature is about 2mm. We describe the center optics as the “water” optics and the annulus as the “air” optics. We tested water optics diameters of 2.0mm and 1.5mm.
Optical modeling conducted on a proprietary human eye optics computer model suggested the use of a variable conic constant as a function of the diameter of the central optics to provide underwater vision. A calculator was programmed to generate the anterior radius and conic constant of the water optics as a function of the air optics radius for the respective eyes, as well as the water optics diameter along with the index of refraction of the selected contact lens material.
Lenses were fabricated by Paragon Vision Sciences in the company’s HDS 100 (paflufocon D) material, using precision polish-free diamond turning, with Optoform 40 computer-controlled lathes. We used the NormalEyes 15.5 fitting system (Table 1), which allows for the selection of three independent zones for the mini-scleral design.
Underwater Clinical Testing
An Institutional Review Board approved a human subjects protocol and informed consent document for the investigation of mini-scleral lenses with two optical powers. The subjects executed the informed consent document. Our first underwater tests were conducted in a freshwater swimming pool with plastic Bailey-Lovie distance and near charts, an occluder, and hand-held trial lenses (Figure 4). The hand-held lenses were calibrated for their power in water (Table 2). The clinical endpoints were as follows:
• Contact lens visual acuity in air
• Contact lens distance and near visual acuity in water
• Distance spherical over-refraction in water
• Blur in and blur out of near chart in water
• Subjective ratings of vision in air and in water
Clinical Observations
The impact of the high-powered near segment on the subjective rating of vision in air was negligible. The visual acuity in air was equivalent to the habitual logMAR acuity of the test eyes. Immediately after immersion in water, subjects reported that their vision in water was excellent. As experienced divers who use scuba masks, the subjects reported an immediate awareness of a full field of view.
TABLE 1 | |||
---|---|---|---|
Parameter Lookup Table for NormalEyes 15.5 Lens | |||
FLAT K OF REGULAR CORNEA | SUGGESTED BCR | SUGGESTED DX RZD | SUGGESTED DX LZA |
Steeper than 48.50 | 7.40 | 1.150 | 60-64 |
47.50 to 48.37 | 7.40 | 1.050 | 60-64 |
46.50 to 47.37 | 7.40 | 0.950 | 60-64 |
45.00 to 46.37 | 7.80 | 1.000 | 60-64 |
44.25 to 44.87 | 7.80 | 0.900 | 60-64 |
42.75 to 44.12 | 8.20 | 0.950 | 60-64 |
41.75 to 42.62 | 8.20 | 0.850 | 60-64 |
40.50 to 41.62 | 8.60 | 0.900 | 60-64 |
Flatter than 40.37 | 8.60 | 0.800 | 60-64 |
Corneal diameter >12.2 increase LZA 2° |
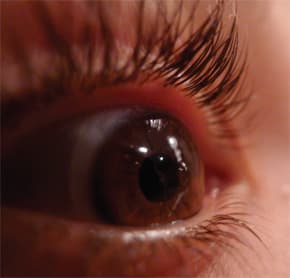
Figure 3. Amphibious lens on-eye.
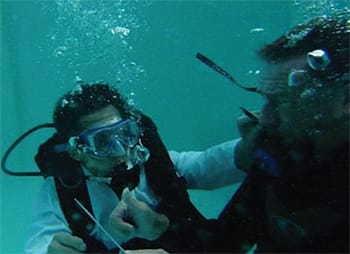
Figure 4. Dr. Chou testing near vision of subject in water using scuba equipment.
Upon testing distance visual acuity, we discovered a hyper- or super-acuity, with underwater logMAR approaching −0.2 (20/12.5) in each of the four eyes wearing the lenses. A subsequent investigation for the basis of the super-acuity provided the explanation based on the optical physics involved. When viewing objects in air, the nodal points of the eye reside at roughly the location of the posterior surface of the crystalline lens. In transitioning to the underwater environment, the water optics maintain the net power of the eye, but the nodal points shift away from the retina to become nearly coincident with the principal planes that lie immediately behind the posterior surface of the cornea. The effect of these shifted nodal points is that the angle subtended by a submerged object becomes larger when viewed with the water optics than when the same object in air is viewed with the air optics of the lens. Theoretically, a logMAR 0.0 (20/20) target in air would appear the same size as a logMAR −0.14 (20/14.5) target with the water optics in water. These theoretical results are consistent with our observations.
TABLE 2 | |
---|---|
Trial Lens Power Corrected for Immersion in Water | |
LENS POWER (AIR) | LENS POWER (WATER) |
±1.00D | ±0.36D |
±2.00D | ±0.73D |
±3.00D | ±1.09D |
±4.00D | ±1.45D |
±5.00D | ±1.82D |
The testing of underwater near visual acuity also revealed super-acuity and an apparent enhanced accommodation and focal range. One subject was fully presbyopic, and the other was prepresbyopic. Both subjects had super-acuity at near that matched their super-acuity at distance and demonstrated a focal range in water (blur in to blur out) that exceeded their range in air with habitual correction. This effect is likely because of the narrow diameter of the water lens, providing a pinhole effect and consequently increasing the depth of field.
The mean and standard deviation for the clinical endpoints are reported as a function of the segment diameter of the underwater lenslet (Table 3). The mean visual acuity of the 2mm-segment lenses in air was 0.05 logMAR when compared with the mean of the 1.5mm-segment lenses of 0.04 logMAR. Although not clinically significant given the coefficient of variation of logMAR testing, it appears the larger lenslet diameter may have an impact on subjective vision in air along with the logMAR acuity in air, while providing greater visual performance in water.
TABLE 3 | ||||||||||
---|---|---|---|---|---|---|---|---|---|---|
Mean and Standard Deviation of Clinical Measures of Four Eyes of Two Subjects With Two Water Optics Lenslet Diameters | ||||||||||
BCLVA (logMAR) | Test lens segment diameter (mm) | Distance CL VA air logMAR | Distance CL VA water logMAR | Near CL VA water logMAR | Spherical over-refraction water | Near blur in water (cm) | Near blur out water (cm) | Subjective vision rating air (1-10) | Subjective vision rating water (1-10) | |
Mean | 0.03 | 1.5 | 0.04 | −0.16 | −0.05 | 0.79 | 19.00 | 93.75 | 8.00 | 9.50 |
StDev | 0.01 | 0.01 | 0.05 | 0.06 | 1.55 | 5.35 | 7.50 | 0.00 | 0.58 | |
Mean | 0.02 | 2.0 | 0.05 | −0.17 | −0.05 | 0.76 | 20.25 | 100.00 | 7.00 | 10.00 |
StDev | 0.02 | 0.01 | 0.04 | 0.06 | 1.76 | 10.40 | 9.13 | 0.00 | 0.00 | |
* Over-refraction reported as the power of lenses in water as per Table 2. |
Discussion and Conclusion
The tested lenses were stable under water and did not dislodge. The subjects attempted extreme gaze excursion and tried to physically rub or move the lenses. Lens stability was consistent with reports of the inherent difficulty of removing mini-scleral lenses (DeNaeyer, 2010). Subjective ratings of vision in air and in water were high. Distance visual acuities in air were equivalent to the subjects’ best spectaclecorrected visual acuities. Of interest is the super-acuity in water at distance and at near along with the enhanced focal range at near.
These amphibious optics contact lenses show promise for applications in which good vision in air and water free from masks or goggles may be needed. Their stability suggests that the lenses would not dislodge with diving or extreme gaze. These lenses could be useful for competitive swimmers, water polo participants, and recreational swimmers. This limited trial suggests that the optics, stability, and protection factors could have utility for war fighters faced with amphibious demands and the need to decrease the size and weight of equipment.
Given the small sample size of our first underwater clinical test of these lenses, further testing with expanded methods and endpoint objectives is needed. Although theoretical models could predict the impact of water pressure changes on the lens-eye relationship, as well as the diffusion of oxygen to the cornea when surrounded by water, actual underwater experimental outcomes are of interest.
Controversy regarding the increased risk of microbial keratitis is likely to overshadow the application of amphibious lenses. However, we believe that there is value in knowing the potential of the design for providing clear vision in air and in water when these optics are placed in a stable GP material and mini-scleral design. CLS
For references, please visit www.clspectrum.com/references.asp and click on document #206.