SAGITTAL HEIGHT
What You Need to Know About Sagittal Height and Scleral Lenses
Understanding the shape of the anterior ocular surface is essential to successful scleral contact lens fitting.
By Lee Hall, BSc (Hons), PhD, MCOptom, FBCLA
Scleral contact lenses have experienced a resurgence in recent years. This is largely due to a better understanding of the corneo-scleral topography and to improvements in lens materials and manufacturing techniques, all of which have paved the way for the development of a new range of innovative and comfortable lens designs.
While scleral lenses are still indicated for ocular surface disease, irregular corneas, post-corneal graft, and post-refractive surgery, they are also becoming increasingly popular for the correction of more “normal” eyes, including those that have high refractive errors and presbyopia, as well as for sporting activities.
Scleral contact lens fitting has traditionally been accomplished by specialist practitioners. Modern scleral lenses are now designed with ease of fitting in mind, which takes much of the guesswork out of the fitting process. However, an appreciation of how the ocular anatomy influences ocular sagittal height, and therefore the scleral lens shape, is essential to the fitting process. This article will review the fundamentals of sagittal height and how it applies to scleral lens fitting.
Scleral Lens Classification
Scleral lenses have previously been classified purely in terms of overall diameter (Sindt, 2008; DeNaeyer, 2010). This approach does not take into account the wide variation in corneal diameter and, consequently, can result in scleral lenses that unintentionally bear on a different surface to that intended when fit on a particularly large or small cornea.
More recently, the Scleral Lens Education Society (SLS) has recommended a classification system (www.sclerallens.org) based instead on the tissue area on which lens bearing occurs (Table 1). Under this system, corneal lenses are defined as those that rest on the cornea only, whereas corneo-scleral lenses extend onto the sclera but are still partially supported by the corneal tissue. Scleral contact lenses, by contrast, are supported entirely by the conjunctival tissue overlying the sclera, with the express aim of completely vaulting the cornea and the limbus.
Lens Type | Description | Definition of Bearing Area |
---|---|---|
Corneal | Lens rests entirely on the cornea | |
Corneo-scleral | Lens rests partly on the cornea, partly on the sclera | |
Scleral | Mini-scleral lens is up to 6mm larger than the HVID | Lens rests entirely on the sclera |
Large scleral lens is more than 6mm larger than the HVID |
A further distinction is made for scleral lenses, with mini-scleral lenses defined as those up to 6mm larger than the horizontal visible iris diameter (HVID) and large scleral lenses defined as those more than 6mm larger than the HVID. For a cornea with an HVID of 11.90mm, this would mean that a mini-scleral lens would be approximately 16mm to 18mm in diameter, whereas a large scleral lens would be approximately 18mm to 24mm in diameter.
For a scleral lens fitting to be successful, the lens should completely clear the cornea and limbus 360º around—without pressure or bearing on either surface—and provide apical clearance sufficient enough to form a fluid tear reservoir beneath the lens and in front of the eye. The lens periphery should also bear evenly on the conjunctival tissue that overlies the sclera to prevent conjunctival redness or blanching of the conjunctival vessels.
In practical terms, this means fitting a lens of greater sagittal depth than that of the ocular sagittal height. Figure 1 shows that the total scleral lens sagittal depth required to fit the eye is ultimately dictated by the sagittal depths contributed by both the corneal and the scleral components of the eye and to the desired amount of apical clearance—i.e., the total ocular sagittal height plus the apical clearance.
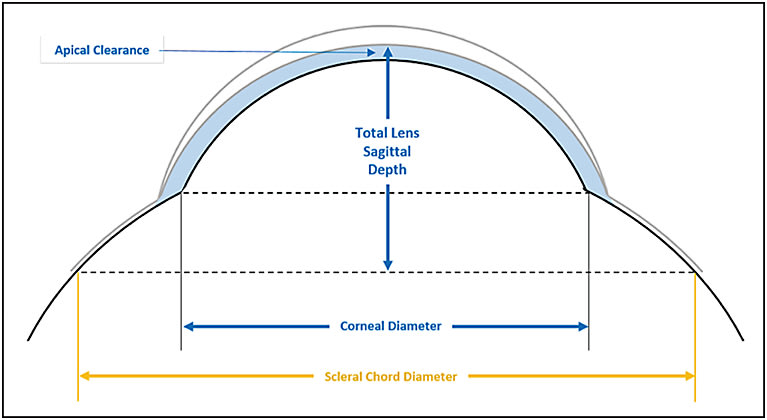
Figure 1. Lens sagittal depth due to sagittal contributions of the cornea, sclera, and apical clearance.
Theoretical Ocular Sagittal Height Considerations
The theoretical ocular sagittal height can be determined mathematically using the formula: Total Ocular Sag = S1 + S2 – S3 (Figure 2), in which S1 and S2 are the corneal and scleral sagittal heights, respectively. The theoretical ocular sagittal height can be calculated using the equation:
in which r = corneal or scleral radius of curvature, p = corneal or scleral shape factor (where p = 1-e2), and d = corneal or scleral diameter, accordingly.
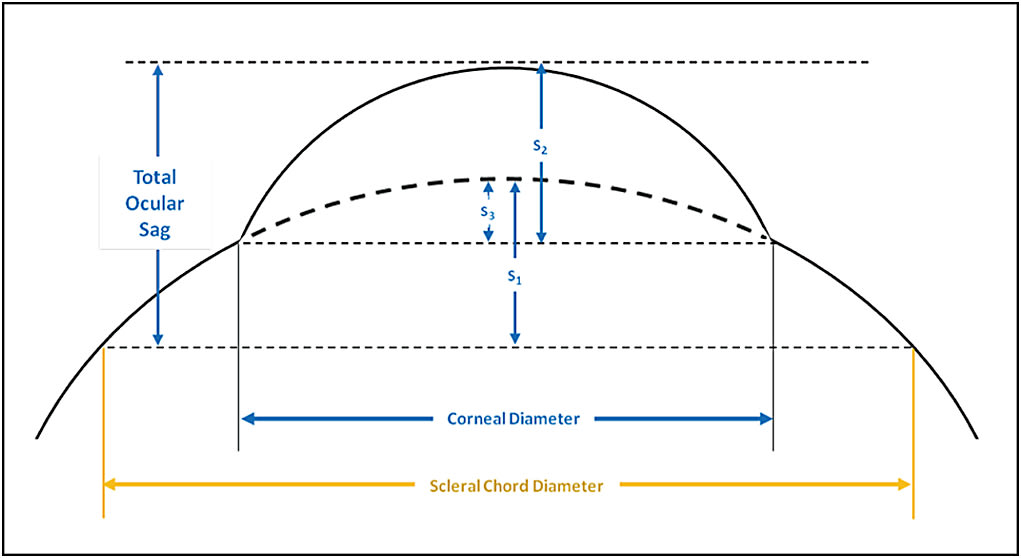
Figure 2. Mathematical model for the calculation of total ocular sagittal height.
Using this model, Young (1992) systematically evaluated the effect of typical variations in the ocular topography on ocular sagittal height. In ranking order, he found that changes in corneal diameter and eccentricity resulted in the most variation, followed in lessening degrees by corneal curvature, scleral radius, and scleral eccentricity.
A “typical” eye with an HVID of 11.90mm, radius of curvature of 7.85mm, and an eccentricity of 0.55 (and assuming a scleral radius of 18.0mm over a 15.0mm chord) would have a theoretical ocular sagittal height of 3,150 microns. A variation in corneal diameter of only 1mm either way results in very different sagittal heights of 2,880 and 3,510 microns for corneal diameters of 10.90mm and 12.90mm, respectively—a difference of approximately 630 microns between the two extremes. Figure 3 shows differences in sagittal height for two corneas of the same radius of curvature but differing corneal diameter.
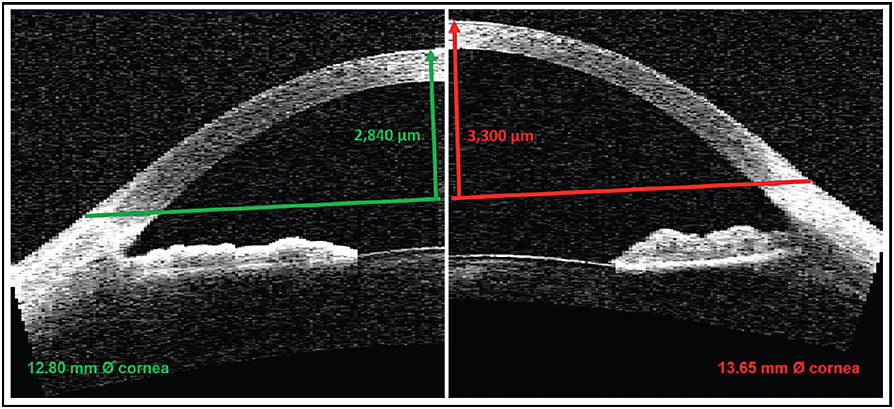
Figure 3. Corneal sagittal height for corneas of the same central radius of curvature (7.85mm), but differing corneal diameters.
Changes in corneal eccentricity have a similar, although slightly less pronounced, effect on ocular sagittal height. For example, substituting eccentricity values of 0.30 (more spherical) and 0.70 (more aspheric) for the “typical” eye described above results in ocular sagittal heights of 3,275 microns and 3,060 microns for the two values, respectively—a difference of 215 microns between the two. While smaller than the changes effected by variations in corneal diameter, the difference in sagittal height is still clinically significant. Interestingly, a variation of approximately 0.40mm in radius of corneal curvature is required to effect a similar change in ocular sagittal height for the same eye.
Variation in corneal diameter clearly has the greatest effect on ocular sagittal height. However, certain combinations of the ocular parameters will also impact the sagittal height; larger corneas of steeper corneal curvature and/or lower eccentricity, for example, will exhibit significantly greater sagittal height, while smaller corneal diameters of flatter corneal curvature and/or high eccentricity will exhibit significantly smaller sagittal height compared to the “average” eye, and such factors should be considered prior to lens selection. Combinations of ocular parameters like these may also partly explain why some eyes, even those considered normal, are more challenging to fit compared to others.
Ocular Sagittal Height in Normal and Irregular Eyes
Although the mathematical model described earlier allows us to predict ocular sagittal height, it assumes an elliptical cornea and a spherical sclera; consequently, it is overly simplistic. Advances in technology have allowed us to more accurately (and more quickly) determine the actual ocular sagittal height.
A number of studies (Hall et al, 2011; Hall et al, 2013; Sorbara et al, 2010; Sorbara et al, 2013), in particular, have provided detailed information about the metrics of the anterior eye. In addition to providing accurate corneal sagittal height data based on corneal diameter, these have also provided data for the total ocular sagittal height taken over a 15.0mm scleral chord (OS15). This is a particularly useful metric as it coincides with the landing zone of a typical scleral lens and provides an approximate indication of the lens sagittal depth required for a given eye.
In a large-scale study examining 204 normal eyes using anterior segment optical coherence tomography (AS-OCT), Hall et al (2013) found a mean corneal sagittal height of 3,170 microns, with a variation of ± 200 microns among eyes and a range of between 2,570 and 3,710 microns. The mean OS15, by contrast, was greater at 3,700 microns, albeit with a smaller variation of ± 170 microns and a smaller range of between 3,230 and 4,080 microns. These findings were almost identical to those of another study (Sorbara et al, 2010), which found an OS15 of 3,740 microns and a similar degree of variation and range among eyes.
Sagittal height varies widely for irregular, diseased eyes. Taking measurements of HVID corneal height and OS15 along the flat and steep keratometry axes, Sorbara et al (2013) compared the sagittal heights of keratoconic eyes and normal eyes. They found that the corneal sagittal height was significantly greater for the keratoconic group of eyes compared to the normal group (3,410 microns versus 2,420 microns). It is important to note, however, that the variation in corneal sagittal height was also much greater for the keratoconic group than for the normal group (880 microns versus 90 microns) and, as might be expected, dependent on the stage of the disease. Likewise, the OS15 was significantly greater in the steep meridian for the keratoconic group compared to the normal group (3,930 microns versus 3,700 microns), as was the variation among keratoconic eyes compared to normal eyes (250 microns versus 160 microns).
Lens Sagittal Depth and Scleral Lens Fitting
Mini-scleral and large scleral lenses have three main components: the central optical zone; the transition zone, which bridges the limbus; and the landing zone, which rests on the sclera. Each zone is composed of one or more curvatures over a given diameter, and each zone, in turn, contributes to the overall sagittal depth of the lens. Scleral lens fitting can be thought of as a process of manipulating the three zones to achieve the required lens sagittal depth relative to the ocular profile, while ensuring even bearing of the landing zone on the sclera.
Various authors have described the use of AS-OCT to determine sagittal height and successfully fit scleral lenses, most notably Gemoules (2008) and Le et al (2012). However, dedicated AS-OCT systems that are capable of capturing the whole cornea and also the sclera for measuring OS15 remain a luxury for most eyecare practitioners. Aside from impression taking, the process of scleral lens fitting today still largely relies on the tried and tested approach of diagnostic evaluation: applying a trial lens onto the eye, assessing the lens fit with the instillation of fluorescein, and modifying the lens as required.
Most laboratories use radii of curvature to specify the base curve and peripheral back curve architecture. This allows for a high degree of customization of the individual zones to optimally fit the ocular profile, although the modification process can sometimes prove a challenge for even the most experienced lens fitters.
Other laboratories specify their lens designs in terms of lens sagittal depth (sag), in which the lenses are typically supplied in 100- or 200-micron steps to achieve the required lens vault. This can make the fitting process much more intuitive, with a lens of greater sagittal depth resulting in a steeper fit, and one with lesser depth resulting in a flatter fit.
That said, many laboratories will readily provide assistance in modifying the lens for even a conventional curvature-based design to achieve the desired sagittal height. Irrespective of whether the lens is specified in curvature or sag, an appreciation of how the lens parameters and zones interact to influence the overall lens height can help in the fitting process.
Central Optical Zone Although keratometry readings provide some idea of the nature of the cornea, particularly for irregular corneas, they don’t provide any information about the required sag. Most corneal topographers (and certainly AS-OCT instruments) will provide sagittal height data, and this can be used as a starting point in selecting an initial trial lens. In the absence of any sagittal height data, a simpler approach is to consider the presenting condition.
The fitting guide of one mini-scleral lens design recommends a first choice lens of 4,200 microns for eyes of “normal” depth that have ocular surface conditions or following refractive surgery. This approximately equates to the mean OS15 values cited previously (i.e., approximately 3,750 microns plus an additional 450 microns for apical clearance). For keratoconic eyes or eyes that have corneal thinning disorders, an extra 200 to 300 microns of lens depth is recommended, with the 4,500-micron lens representing the first choice for this design. Finally, a 4,800-micron lens is recommended in cases of particularly high corneal grafts.
The first task in assessing the fit of any scleral lens is to evaluate the fluorescein pattern over the central optical zone. In each case, regardless of how irregular, tilted, or protruding the cornea is, the aims are to completely vault the cornea and limbus so as not to cause bearing. And, especially in the case of ocular surface conditions, to form a tear reservoir between the lens and cornea for further protection and relief from symptoms. If any touch is evident, the lens depth should be increased by approximately 300 to 400 microns.
Once the new lens has settled, the corneal clearance can be assessed using a slit lamp optic section to compare the tear lens thickness with either the known lens thickness or the estimated corneal thickness. Alternatively, an AS-OCT device can be used to determine the tear reservoir thickness objectively.
A lens with too great a sagittal depth may result in excessive clearance, with bubbles forming on, or soon after, application and a reduction in visual acuity. A lens with too little a sagittal depth may result in corneal touch and/or bubbles in the limbal area. In either case, a lens with either a lesser or greater sag should be tried accordingly.
There is considerable debate as to what constitutes the optimal apical clearance between the cornea and the lens back surface, including the impact on corneal physiology. Scleral contact lenses have considerably greater center thicknesses, and therefore decreased oxygen transmissibility characteristics, compared with corneal GP lenses. Further, the lens tear reservoir can also act as a barrier to oxygen flow. For this reason, practitioners should aim for an initial apical clearance of approximately 400 microns to achieve a post-lens tear thickness of between 100 to 250 microns (Michaud et al, 2012) following the settling that inevitably occurs as the lens sinks into the conjunctiva.
The central optical zone fit can be changed by altering the overall lens sagittal depth, as described previously, and by any adjustments in peripheral fit made after the optimum vault is achieved. Occasionally, the vault may be optimal, but the central optical zone diameter is either too large or too small. In such cases, the central optical zone diameter should be increased while flattening the lens base curve, or decreased while steepening the base curve, to maintain the same degree of vault. The lens power should then be adjusted accordingly to compensate for the change in base curve radius of curvature.
Limbal Transition Zone Once a suitable central zone fit has been established, the limbal transition zone should be assessed. The aim is to completely vault the limbus through 360º to avoid any insult to the limbal tissue; an optimal fit will show an even band of fluorescein “bleeding” out over the limbus and tapering out just onto the sclera.
Relatively little was known about the limbal profile and its contribution to ocular sagittal height until recently. Meier (1992) previously defined the corneo-scleral profile (CSP) based on observations of the limbal zone made using the naked eye or a slit lamp. He described five different corneo-scleral transition models (Figure 4): a gradual transition from cornea to sclera, in which the scleral portion is either convex (Profile 1) or tangential (Profile 2); a marked transition, in which, again, the scleral portion can be either convex (Profile 3) or tangential (Profile 4); and finally, a fifth, sinusoidal profile, in which the convex cornea blends into a concave sclera (Profile 5). Interestingly, the profiles decrease in sagittal depth across the classification, with Profile 1 exhibiting the greatest sagittal height and Profile 5 the smallest.
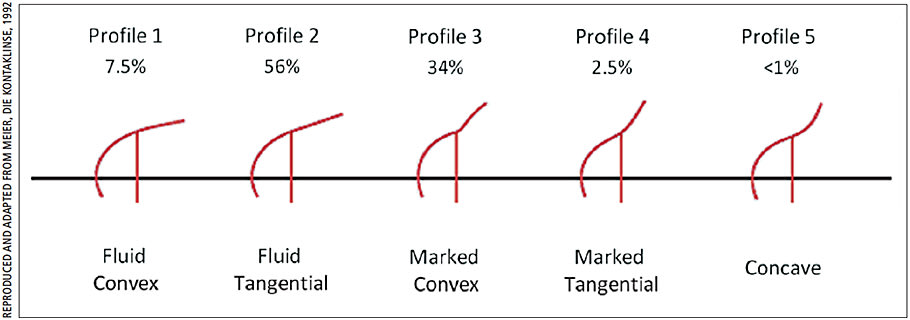
Figure 4. Classification of corneo-scleral transition profile, according to Meier.
Meier’s assessments of CSP were limited to the superior corneo-scleral junction, and a subsequent study showed that this was neither an accurate nor a reproducible means of classifying the CSP (Bokern et al, 2007). A more recent study by Hall et al (2013) that assessed the superior, inferior, nasal, and temporal junction angles, however, found that 77% of all transition angles were within ± 5°of 180°, demonstrating an almost tangential extension of the cornea to form the paralimbal sclera in these cases.
Manufacturers are increasingly incorporating the corneo-scleral topography into their lens designs for improved comfort and fit, with limbal zone adjustments increasingly made in terms of tangents rather than curvature. For example, some designs utilize a tangent angle approach for adjusting the limbal zone, offering either incremental changes in the tangent angle or several different tangent profiles.
Some lens designs incorporate reverse geometry elements to improve the fit in this area. In addition, designs are increasingly becoming available with a choice of prolate or oblate geometries, with the latter intended to improve the fit in post-graft, post-refractive surgery, or corneal marginal degenerations.
Landing Zone The final assessment of scleral lens fit relates to the landing zone. The aim here is to spread the lens bearing evenly and over as broad an area of the sclera as possible so as to avoid compression of the conjunctiva and its blood vessels.
Too steep a landing zone can result in an overly tight lens fit, further resulting in conjunctival redness, vessel blanching, or even conjunctival prolapse. In such situations, the bearing surface is also displaced away from the limbus and may unintentionally cause the lens to vault, resulting in an unwanted increase in apical clearance. These effects can be alleviated by flattening the peripheral curves in a conventional design or by making adjustments to the scleral zone tangent angle in newer designs that allow this. Conversely, too flat a landing zone can result in excessive edge lift and decreased comfort, which can be corrected by steepening the peripheral curves or the scleral zone tangent angle.
Any alteration in curvature or tangent angle in either the limbal transition zone and/or the scleral landing zone will inevitably result in a change in overall lens sagittal depth, and this should be compensated for in the central optical zone. However, for some lenses, the lens design itself or the manufacturer will compensate for any curvature or tangent angle changes so that the revised lens will maintain the same sagittal depth fitting relationship with the ocular profile.
Various studies have highlighted the scleral profile’s asymmetric nature (Hall et al, 2011; Hall et al, 2013; van der Worp et al, 2010). A recent study by Ritzmann et al (2015) at Pacific University evaluated the scleral profiles of 40 normal healthy subjects (80 eyes) using the Eye Surface Profiler (Eaglet Eye), an instrument capable of imaging the sclera to a width of up to 20mm. Measurements of scleral sagittal depth, referenced from the apex of the cornea, were taken in the primary and oblique positions of gaze at chords of 13.0mm, 15.0mm, and 17.0mm. Their results showed marked asymmetry 360º around the scleral faces, but also marked differences in sagittal depth between opposing faces in the horizontal and vertical meridians, with the nasal and superior faces exhibiting the least scleral sagittal depth (i.e., protruding anteriorly the most) in each case (Figure 5). Importantly, however, the disparity increased significantly with the increase in chord diameter, especially along the horizontal meridian.
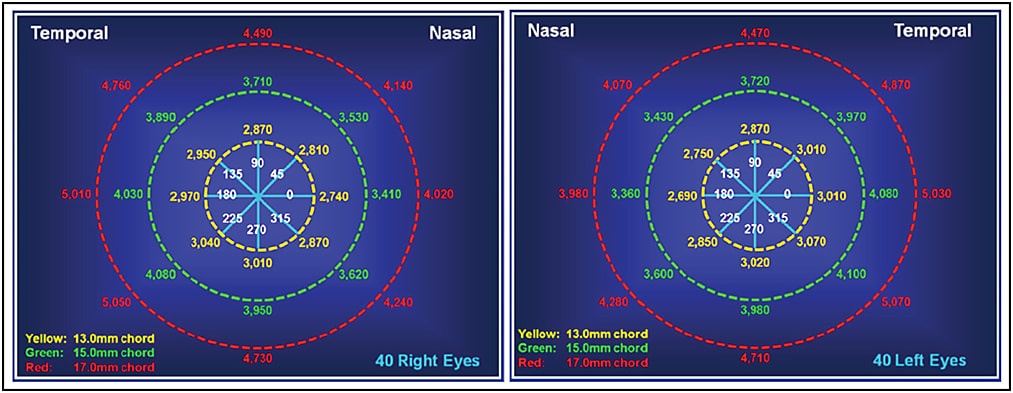
Figure 5. Summary of mean scleral sagittal depths at chords of 13.0mm, 15.0mm, and 17.0mm for right and left eyes.
This accounts for why many scleral lenses are prone to inferior temporal decentration, as the areas of least scleral depth (nasal and superior) are where the scleral lens will “land” first (Figure 6). The lens will search for its resting point of equilibrium and move in the direction of greatest depth, the areas of least mechanical resistance, with a subsequent shift inferio-temporally.
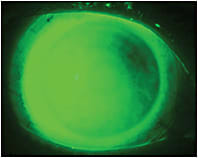
Figure 6. Scleral lens bearing nasally and superiorly due to asymmetry in scleral sagittal height.
The highly asymmetric nature of the sclera highlighted in this study also accounts for why it is not always possible to fit an eye with a symmetrical lens design. In such cases, a symmetric lens may cause unacceptable impingement in one or more regions of the sclera, and a nonrotationally symmetrical peripheral lens design, with a toric or quadrant-specific geometry, may be required to facilitate an acceptable fit.
Conclusion
Sagittal height is the basis for successful scleral lens fitting. An appreciation of how the ocular anatomy influences ocular sagittal height, and therefore the lens shape, is essential to the fitting process. CLS
For references, please visit www.clspectrum.com/references and click on document #234.
![]() | Dr. Hall works for Visioncare Research, an independent research organization to the eyecare industries. He is also a Visiting Research Fellow at Aston University, Birmingham, in the United Kingdom. He has received research funding from Johnson & Johnson, Alcon, and CooperVision. |