CORNEAL CROSS-LINKING
Corneal Cross-Linking: Past, Present, and Future
With FDA approval on the horizon, learn the history of this procedure and where the technology is headed.
By Clark Chang, OD, MSA, MSc, FAAO
Biomechanical weakening in keratoconus and postoperative ectasia results in a pathognomonic conical corneal protrusion and corresponding optical irregularities, which may serve as a reflection of disease severity. Therefore, progressive visual impairment ensues over time due to accompanying induced irregular astigmatism, higher-order aberrations, and stromal scarring (Rabinowitz, 1998; Alió and Shabayek, 2006). Because conventional spectacle lenses often fail to achieve functional improvement, specialty contact lenses are typically required for visual rehabilitation. However, as the keratoconus disease stage advances, generally from the second through the fifth decade of life, contact lens fitting challenges and/or intolerance may emerge.
This disease cycle, once widely perceived as clinically inevitable, necessitates penetrating keratoplasty (PKP) in 10% to 20% of cases (Rabinowitz, 1998; Gordon et al, 2006). Undoubtedly, the PKP graft survival rate has consistently improved secondary to new donor tissue preservation techniques and to new-generation pharmacological agents and surgical instruments, such as femtosecond lasers; nonetheless, many patients continue to report dissatisfaction with their post-operative visual outcomes.
A Changing Paradigm in Keratoconus Management
While the conventional medical management model for keratoconus focuses on optical rehabilitation until such need for PKP arises, the contemporary management paradigm now includes additional components such as earlier diagnosis and disease stabilization (Hersh et al, 2011).
In recent years, corneal collagen cross-linking (CXL) has emerged as a promising management option for keratoconus and corneal ectasia. CXL is currently an investigational procedure in the United States, but it has been available outside of the United States for a number of years. It has been shown to successfully—with clinical reproducibility—stabilize progressive corneal ectatic disorders, and it has a good safety profile (Chang and Hersh, 2014; Hashemi et al, 2013; Wollensak et al, 2003; Hersh et al, 2011). In CXL, the interaction of ultraviolet-A (UVA) light (365nm) and riboflavin molecules leads to the formation of cellular “cross-links” within the collagen and intracellular matrix of the stroma, resulting in stiffening of the corneal stroma, most predominantly in the anterior 300 microns (Spoerl et al, 2007).
While the primary goal of CXL treatment is to slow down or halt further disease advancement, many investigators have also reported clinically significant improvements in postoperative topographic, aberrometric, refractive, and visual outcomes in some subjects (Chang and Hersh, 2014; Hersh et al, 2011; Vinciguerra et al, 2009). Because of these positive clinical findings in the literature, combined with its relatively low complication rates, CXL has become widely accepted as one of the frontline treatment options for progressive keratoconus and postoperative ectasia.
Notwithstanding, continual keratoconus progression and vision loss have also been observed after CXL (Raiskup et al, 2015; Caporossi et al, 2013); therefore, further long-term studies are needed to determine specific risk factors that may be correlated to treatment complications. Thus, it is still advisable for eyecare providers to closely monitor patients after CXL despite a low rate of treatment failure reported with the conventional CXL treatment involving removal of epithelium.
Given the safety profile and low complication rates described thus far, clinicians may now help patients maintain or improve their quality of life by preventing progression, which may also enable these patients to defer or potentially eliminate the need for PKP over their lifetime (Hashemi et al, 2013).
The Dresden Protocol: Where It All Started
Photochemical-activated cross-linking has long been utilized to change material biochemical characteristics during dental surgeries and tissue transplantations. Moreover, it is also a normal physiological process that is time-dependent in all collagen-based systems to slowly increase tissue rigidity with age—a suspected contributing reason for the relative keratoconus stability in older patients. It was introduced as a novel ocular procedure by Wollensak et al (2003) to increase corneal stiffness in keratoconic corneas by approximately 300%; it later became known as the Dresden Protocol or “epi-off” CXL. In this procedure, a photosensitizer (riboflavin in Dextran T500 solution) is exposed to UVA (365nm) irradiation that likely results in the production of free singlet oxygen radicals and induces formation of new cross-links. Although these cross-links were first theorized to occur at inter- or intra-fibrillar collagens, the exact locations for these new linkage sites are still being investigated; some investigators now report that these covalent cross-links are created between collagen and glycosaminoglycan molecules (Hersh, 2015).
Due to the presence of epithelial tight junctions, achieving desirable stromal riboflavin concentration was perceived as challenging because of the macromolecular and hydrophilic characteristics of riboflavin-Dextran; therefore, it was regarded as essential by the Dresden Protocol to first remove epithelium prior to CXL treatment. Typically, when performing the Dresden Protocol at our center (Cornea and Laser Eye Institute-Hersh Vision Group), epithelial debridement is conducted over the central 9mm zone after administration of topical anesthesia. Over the next 30 minutes following epithelial removal, topical riboflavin is instilled every two minutes. At the conclusion of this loading phase, stromal riboflavin saturation is confirmed via slit lamp examination (Figure 1).
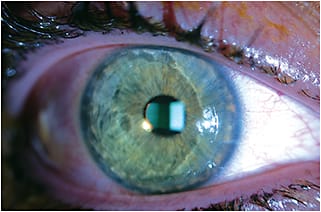
Figure 1. Anterior chamber evidence of corneal riboflavin saturation in an epi-off CXL case.
In addition, a minimum pachymetric reading of 400µm is confirmed via ultrasound pachymetry prior to the UVA irradiation phase to ensure ocular safety. This is considered necessary because 85% to 90% of UV energy dissipates in the anterior 400µm of the cornea, thereby reducing the energy threshold to approximately 0.18 J/cm2 at the level of the endothelium, which is about half of the endothelial damage threshold. With sufficient corneal tissue thickness confirmed, the corneal treatment zone can then be exposed to a UVA source emitting a selective wavelength of 365nm at an irradiance of 3.0 mW/cm2. Riboflavin drops continue to be administered every two minutes during this 30-minute UVA exposure phase to allow continuance of the clinically controlled photochemical cross-linking that totals an energy output of 5.4 J/cm2.
At the end of each treatment, antibiotic and corticosteroid drops are instilled prior to the placement of a therapeutic bandage lens. The therapeutic lens is removed within three to five days, pending epithelial healing. In addition, topical antibiotic and corticosteroid usage are prescribed for one week and two weeks, respectively, in the postoperative period.
Setting Patient Expectations: Early Postoperative Trends
Recent epidemiology studies in different parts of the world suggest keratoconus prevalence to be higher than one in every 2,000 as conventionally perceived, which likely reflects the limitations of previous diagnostic instrumentation. Keratoconus prevalence in various Asian population groups has been suggested to be much higher compared to those of their Caucasian counterparts; prevalence rates of up to 2.5% have been reported (Hashemi et al, 2014). Undoubtedly, more epidemiology investigations are needed to form a new consensus on an updated keratoconus prevalence rate in the general population or even in different population subgroups. It is known that CXL has been offered as one of the first lines of treatment under the new management model, and clinicians will encounter more patients who have undergone CXL treatment, especially given today’s ease of global mobility. Therefore, familiarity with post-CXL natural clinical courses will facilitate proper patient care and monitoring.
A few meaningful clinical parameters are often used in documenting keratoconus progression and corneal health. Some examples include keratometry, visual acuity, and tissue thickness and transparency—such as the presence or absence of corneal haze or scarring. Our center has found noteworthy trends up to 12 months after epi-off CXL, and we have widely published our body of findings (Chang and Hersh, 2014; Hersh et al, 2011; Greenstein et al, 2010; Greenstein et al, 2011). The aforementioned four clinical variables will be further discussed in this section, as these normal findings are often misinterpreted as postoperative complications by co-managing eyecare providers.
Corneal Haze Cases of CXL-associated stromal haze have been widely noted in the literature. In our clinic we have documented stromal haze in more than 90% of treated eyes in our initial cohort of those treated with epi-off CXL. Post-CXL haze is different from photorefractive keratectomy (PRK) haze in that it extends to the anterior stroma and has more of a dust-like distribution pattern rather than being more superficial (sub-epithelial) and reticulated as with PRK. Using our Scheimpflug camera, we were able to detect a significant increase in corneal haze between baseline and one month. Such cases of haze tend to plateau between one and three months and then decline thereafter (Figures 2 and 3). However, no statistical correlations were found between the degree of haze and postoperative visual acuity, as most clinicians would expect. Thus, the clinical implications of corneal haze as a positive or negative post-CXL indication may need to be further explored in future investigations.
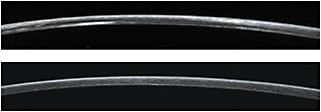
Figure 2. Post-CXL corneal haze comparison via Scheimpflug images between one month (top image) and 12 months (bottom image) after treatment.
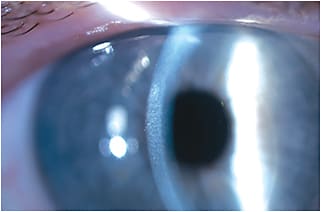
Figure 3. 1+ corneal haze as seen with the slit lamp.
Corneal Pachymetry Corneal thinning has been widely utilized as a defining marker for keratoconus advancement. Hence, it is worth noting that further reduction in corneal thickness has been reported after CXL. This is most likely because corneal lamellae become more compact in arrangement as corneal biomechanical properties improve (Bottós et al, 2008). In our study cohort, statistically significant corneal thinning can be observed between baseline and three months, with subsequent increase in pachymetric values after the three-month’s study time point; however, the mean corneal thickness still remains mildly decreased at 12 months relative to baseline, possibly due to the aforementioned mechanism of action (Figure 4).
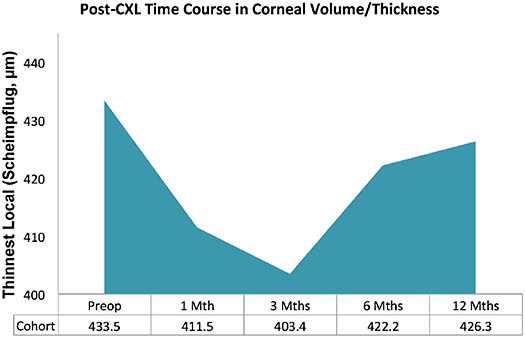
Figure 4. Post-CXL changes in corneal volume over 12 months.
Visual Acuity A decrease in visual acuity (VA) may not always accompany keratoconus progression, but such functional reduction continues to have great clinical relevance in both subjective and objective monitoring. From our data analysis, worsening in both uncorrected and best-corrected VA (UCVA/BCVA) can be observed between baseline and one month. Thereafter, improvement in both variables continues for up to six months and stabilizes after that. At 12 months, improvement of slightly more than one Snellen line is seen in mean BCVA (Figure 5).
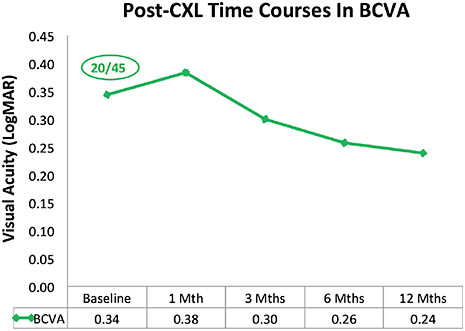
Figure 5. Post-CXL changes in BCVA over 12 months.
Keratometry Similar to the above three clinical parameters, keratometric values are commonly utilized as a measurement tool for keratoconus stability and staging. It is therefore interesting to see a significant steepening in Kmax using a rotating Scheimpflug camera system between baseline and one month, which possibly can be misinterpreted as CXL treatment failure. However, this is succeeded by continual keratometric flattening thereafter for up to six months, with no statistical difference between six months and our study end point of 12 months. Overall, there was a mean 1.7D reduction in Kmax at the 12-month time point.
Epithelium and CXL: The New Clinical Frenemy
The minimum of 400µm of apical thickness for CXL excludes a segment of keratoconus patients who can otherwise benefit from the availability of such a treatment. Further, the removal of epithelium during epi-off CXL has also raised clinical concerns of potential postoperative pain, delayed epithelial healing, infection, inflammation, endothelial decompensation, persistent haze, and scarring. Although epi-off CXL has been reported to have an excellent safety profile, concerns for potential complications can be reduced if epithelial barrier functions are not disturbed during CXL. Transepithelial CXL (TE-CXL) application, a.k.a. “epi-on CXL,” can also serve to expand safety guidelines, even for those who have thinner corneas.
Wollensak and Iomdina (2009) reported a five-fold reduction of CXL efficacy if the epithelium is preserved prior to CXL treatment. However, newer generations of riboflavin formulations that contain Dextran solvent substitutes, higher riboflavin concentration, and/or additional corneal enhancers, such as benzalkonium chloride, edetate disodium (EDTA), and tromethamine (TRIS), have proven to have better stromal uptake even without full epithelial debridement (Figure 6). In addition, longer pre-operative riboflavin loading time (45 to 60 minutes) and the resultant higher stromal level of riboflavin bioavailability have also served to improve efficacies of TE-CXL.
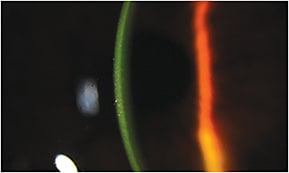
Figure 6. Observance of riboflavin penetrance following TE-CXL.
COURTESY OF ANDREW MORGANSTERN, MD
Although many comparative reports still show a shallower treatment depth with intact epithelium via TE-CXL, it is still undetermined how much CXL effect is needed for keratoconus stabilization; it is possible that TE-CXL offers sufficient improvement in corneal rigidity for most keratoconus patients, especially as the technique evolves in the future. Recent reports have suggested higher rates of keratoconus progression at 10 to 24 months after TE-CXL (Caporossi et al, 2013; Soeters et al, 2015); thus, close patient monitoring to identify the potential need of CXL retreatment is still recommended. Regardless, the availability of TE-CXL has tremendous clinical benefit for those keratoconus patients who may not qualify for epi-off CXL.
You Can’t Hurry Love, But Maybe CXL
The relatively long CXL treatment duration (riboflavin loading + UV irradiation phases) can be challenging for patients who are less cooperative despite their own best effort. For example, it may be challenging for pediatric patients to remain comfortable throughout the entire intraoperative time period. Hence, patient comfort and experience can be greatly improved with a less time-intensive CXL protocol.
The accelerated CXL (aCXL) protocol has been made possible by applying the principle of the Bunsen-Roscoe Law of Reciprocity. This fundamental law of photochemistry dictates that the effects of photochemical activation and subsequent biological polymerization are proportional to the total energy output delivered regardless of the applied UV irradiance and treatment time. Simply put, the CXL energy dose delivered to a given cornea is kept at a constant threshold provided that the combined products of the UV exposure time and illumination dose are equal.
So, the aCXL protocol theoretically delivers the same total amount of energy at 5.4 J/cm2 despite utilizing a 10-fold increase in fluence level (30 mW/cm2) provided that the treatment duration is reduced to only three minutes (rather than 3 mW/cm2 in 30 minutes, as in the Dresden Protocol). Tomita et al (2014) performed these two described protocols to compare treatment effects; the authors reported that both procedures are effective in stabilizing keratoconic eyes at 12 months. Moreover, optical coherence tomography (OCT) also revealed similar treatment depth in both epi-off CXL and aCXL groups, which shows deeper tissue penetration than that demonstrated by TE-CXL. Nonetheless, given that epithelium was removed in both treatment arms, this OCT finding is of no surprise. Although Tomita et al (2014) recognized that a longer follow-up period is needed to fully assess whether differential efficacies exist between the Dresden Protocol and aCXL, they concluded that aCXL offers the additional benefit of shorter treatment duration (Figure 7).
A video showing an aCXL procedure with a 7.2 J/cm² UV treatment dose delivered over four minutes, courtesy of Cosimo Mazzotta, MD, PhD (Siena University Hospital, Italy), is available here.
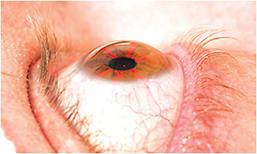
Figure 7. A patient undergoing accelerated CXL.
COURTESY OF AVEDRO, INC.
CXL and LASIK: A New Pairing
Up until now, the goal with CXL has been to revolutionize the management paradigm for keratoconus and ectasia patients. The development of aCXL, nonetheless, has quietly expanded its clinical application. Without the concerns of prolonging the intraoperative time period for laser vision correction surgeries, candidates who are conventionally regarded as having higher risks of a less predictable treatment outcome may now also have improved safety and optical stability after laser-assisted in situ keratomileusis (LASIK) or PRK. These candidates can include younger patients who have a high degree of ametropia and/or astigmatism, patients who have thinner corneas and require higher degrees of correction, or patients who have somewhat intriguing topographical findings without any definitive contraindication.
Excimer ablation with LASIK or PRK presents a direct opportunity for brief applications of riboflavin to the residual stromal bed. A new Dextran-free formulation with a higher riboflavin concentration allows for accelerated riboflavin saturation (45 seconds) in the residual stromal bed. After rinsing off excess riboflavin solution and debris, the stromal bed is exposed to an additional 45 seconds of UV illumination at an elevated fluence level of 30 mW/cm2. This exposes treated eyes to an energy level of 1.35 J/cm2, which is lower than the irradiation output during a keratoconus CXL session (5.4 J/cm2). International studies of such combination procedures have shown adjunctive CXL to result in better visual acuity and refractive predictability without adverse events (Tan et al, 2014; Kanellopoulos and Asimellis, 2014) (Figure 8).

Figure 8. Diagrammatic illustration of LASIK Xtra.
COURTESY OF PETER HERSH, MD
An Exciting Future
With CXL as part of our armamentarium, eyecare professionals are no longer restricted by the traditional tenets of managing optical corrections in keratoconus patients while waiting for the inevitable decline in visual function and possible need for corneal grafts. We may be able to greatly reduce or potentially eliminate the need for PKP in the future by combining early disease detection with ever-improving diagnostic instrumentation and progression prevention via CXL (Hashemi et al, 2013). Additionally, this new management model may also help to improve quality of life as patients may enjoy better functional outcomes with spectacles and contact lenses.
In recent years, CXL has become an essential part of the standard of care for keratoconus patients; it is with excitement that we anticipate U.S. Food and Drug Administration approval of this procedure as well as continual refinements in future CXL protocols to realize its full protective benefits for both irregular and regular cornea patients. CLS
To obtain references for this article, please visit http://www.clspectrum.com/references and click on document #238.
![]() | Dr. Chang is director, Specialty Contact Lens Service at Cornea and Laser Eye Institute-Hersh Vision Group in Teaneck, N.J. He is president of the New Jersey Academy of Optometry as well as an advisory board member of the International Keratoconus Academy for Eye Care Professionals (IKA), the Optometric Council on Refractive Technology (OCRT), and the Gas Permeable Lens Institute (GPLI). Dr. Chang is a subinvestigator in many ongoing clinical trials and has published multiple articles on keratoconus and specialty contact lenses. He is a consultant or advisor to Allergan and Oasis Medical and has received travel funding from SynergEyes. You can reach him at cchang@vision-institute.com. |