MYOPIA CONTROL
Defining a Strategy for Myopia Control
A systematic approach can help practitioners more effectively implement myopia control into practice.
By Langis Michaud, OD, MSC, FAAO (Dipl), FSLS, FBCLA; Patrick Simard, OD, MBA, MSC, FAAO; & Rémy Marcotte-Collard, OD
Any eyecare practitioner who has not been living on another planet for the last few years should by now be aware that myopia is no longer considered a benign refractive error that can be compensated for with regular glasses or contact lenses. In reality, thanks to the tremendous legacy of the late Prof. Brien Holden, we are now aware that myopia has become epidemic and sight-threatening.
In fact, he and other prominent researchers have shown us evidence that high myopia must be now considered an important risk factor for developing significant ocular pathologies leading to visual impairment, such as glaucoma, maculopathy, or retinal detachment. This concerns every myope, not only the most severe cases.
As eyecare practitioners, we treat eye diseases, but we also have to proactively prevent them if we can. This is why we no longer recommend extended wear to anyone wearing low-Dk hydrogel contact lenses, and we caution against the use of tap water in contact lens care to reduce the potential for protozoan and pathogen contamination. By the same token, we should develop and implement a myopia control strategy (MCS) in our practices.
That being said, the next questions are obvious: Who are appropriate candidates? At what stage should we intervene? Which are the correct measures for effectively controlling myopia? Is orthokeratology (OK) for everybody? Can OK increase myopia in some patients? What is the minimal add power necessary to control myopia?
This article attempts to establish these parameters using evidence-based knowledge. Despite the extensive information in this area, we find that the more we know, the more we realize how little we know.
Myopia and Its Impact
As we all are aware, myopia is a refractive error characterized by difficulty in seeing clearly at a distance. More interestingly, for our purposes, we should view myopia as the result of the eye’s failure to develop normally so as to balance the optical power of the eye with its length. We must also treat it as a significant risk factor for visual impairment.
How important is myopia in our industrialized societies? It is generally accepted that the prevalence of myopia has increased around the world in the last two decades. Asian countries are showing the highest rates of myopia and severe myopia, albeit with significant variations from country to country. For instance, in Hong Kong, 18.3% of 6-year-old children are myopic, and 0.7% are already highly myopic. By age 12, those figures reach 61.5% and 3.8%, respectively (Lam et al, 2012). In Taiwan, myopia prevalence surpasses 80% by 18 years of age, affecting females more than males (Guo et al, 2012). In Korea, 96.5% of young adults (19 years of age) are myopic (Pan, Dirani et al, 2015).
In Europe, there has been a significant increase in the prevalence of myopic young adults: 47.2% of 25 to 29 year olds, compared to 20% for this group a few decades ago (Williams et al, 2015). Finally, in the United States, national surveys indicate that myopia appears to have been substantially higher in 2004 compared to 30 years before, affecting 41.6% of the population between 12 and 54 years of age, with 2.2% being highly myopic. Everyone appears to be affected by the increase: African-American and white, male and female (Vitale et al, 2009).
Why should we be concerned? It is known that myopia can represent a significant risk factor in the development of ocular pathology, such as early onset cataract (3x greater risk), myopic macular degeneration and retinal holes and tears (8x greater risk), and glaucoma (18x greater risk) (Tano, 2002). The Blue Mountain Eye Study showed that this risk is not linear but is related to the level of refractive error, starting at 0.3% for very low myopia (–1.00D) and rising to 3.0% for moderate myopia (−3.00D to −5.00D), 28.6% for high myopia (–7.00D to –9.00D), and 52.4% for very high myopia (> –9.00D) (Saw, 2006). In fact, for every –0.25D myopia increase, this risk becomes 17% higher. Severe vision loss is mostly secondary to choroidal neovascularization, which affects 0.1% to 0.5% of myopic patients in Europe and 0.2% to 1.4% of myopic patients in Asia (Wong et al, 2014).
Considering the recent evolution in myopia, its increased prevalence around the world, and its potential to alter visual function as it evolves, it is quite easy to predict that, if nothing is done, myopia will become the major cause of blindness around the world. This is already the case in some countries (Holden et al, 2014).
Consequently, implementing an effective MCS would limit the natural evolution of myopia and thereby reduce the burden and the social costs of vision loss. To achieve this goal, we need to gain a better understanding of the etiology of myopia and of the factors influencing its progression over time.
Where does myopia come from? If this question had been put to Charles Darwin, he would have said that a myopia epidemic would be a natural process of adaptation to our highly scholarly, technology-oriented world. And, in part, he would be correct. In fact, myopia is multifactorial in origin; it is rooted in genetics, environmental conditions, and severe distortion of visual inputs.
Genetics play a role for a small proportion of myopia cases. These cases are clearly considered as “familial” and are characterized by a high level of myopia, a very early onset, and traceable chromosomal locations (Morgan and Rose, 2005). For those who acquire myopia during childhood, genetic factors are more difficult to identify as contributors. Despite the fact that the odds of developing myopia increase from 0.28 with no myopic parents to 0.84 if both parents are myopic (Wu and Edwards, 1999), this type of inheritance is not considered a good measure of genetic background (Morgan and Rose, 2005).
Gene expressions or their mutations are influenced by environmental conditions. Once expressed, they influence biochemical pathways that drive the natural growth of the eye as they do for other biological processes (Feldkämper and Schaeffel, 2003). This explains the process of emmetropization (Wildsoet, 1997). Environmental conditions influence the axial length growth as well as the expression of some genes, while suppressing others. For example, putting filters in the form of positive or negative lenses in front of an animal’s eyes can influence both the way the eyes grow and the development of refractive errors and amblyopia (Schaeffel and Howland, 1991).
Between genetics and the environment, there is strong evidence that the higher prevalence of myopia is the result of adaptation to rapid and environmentally induced change associated with increased education and urbanization (Wojciechowski, 2011). In recent generations, environmental influences have increased dramatically (Wu and Edwards, 1999). To reinforce this finding, a study showed that several ethnic groups exposed to the same environmental conditions ended up with the same level of myopia, despite significant genetic differences (Pan, Chen et al, 2015). Thus, any effective MSC strategy must consider environmental aspects more than genetic aspects.
Notwithstanding their genetic background, it was suggested that emmetropic children are at risk of becoming myopic when their axial length is greater than 23.5mm (Mutti et al, 2007). However, the ratio between axial length and corneal radius (in mm) seems to be a better predictor of myopia onset if it is > 3mm in children aged 6 to 12 (He et al, 2015).
To understand this relationship, it is crucial to consider the importance of the crystalline lens as an essential piece of this complex puzzle. During the emmetropization process, the lens tends to become thinner and flatter and to lose power. In children who will develop myopia, this adaptive process is compromised. In contrast, the cornea remains stable by the age of 3 years. This is why the axial lens/corneal radius ratio increases in myopic candidates and becomes highly predictive of future myopic evolution (Mutti et al, 2012).
Elements Required for an Effective MCS
Based on the discussed definition of myopia, we must consider a number of important elements: the elongation of the eye, which is the primary factor in the occurrence of ocular pathology in high myopia; corneal power; and lens adaptation/power, which is related to the previous two elements.
Another important feature to include in this model is pupil diameter. It drives the optical power of the eye and, eventually, its adaptation. Consequently, it should be part of the discussion.
The environmental factors that should be part of the equation are not only those related to optical stimulation of the eye but also to the conditions surrounding visual tasks. The amount of near vision work, outside activities, and lighting are some of the factors that are known to have a potential impact on the myopia outcome.
Who Are the Candidates?
As a conservative approach, an MCS should be offered to, and implemented for, every patient who is at significant risk of developing progressive myopia and/or in those who present a faster progression rate than expected. As a proactive approach, the issue should be raised with patients who are stable and/or evolving more slowly than average and with those who, while not yet myopic, are showing refractive changes and/or physiological variations predictive of myopia onset.
Tracking Myopia Onset A high variation (0.75D to 1.00D) of refractive power, specifically decreased hyperopia, occurs up to two years before myopia onset (Xiang et al, 2012). This normally occurs when a patient is between 6 and 12 years of age. This rapid refractive change is considered one of the strongest predictors of myopia occurrence (Zadnik et al, 2015).
When myopia becomes clinically visible, the rate of refractive change is reduced (–0.50D to –0.75D per year) and continues to slow over time (–0.25D to –0.50D per year). Following the same pathway, axial length (AL) elongation accelerates in the year before myopia onset. As already stated, the AL/corneal radius ratio is normally > 3mm at that time.
It is crucial to keep in mind that myopia progression is not linear. There are periods of rapid evolution, followed by apparent stabilization. It would be preferable for a practitioner to evaluate cases using a long-term perspective rather than managing patients based on a narrow timeline.
Developing a Strategy
When implementing myopia control into practice, it helps to have a systematic approach for the management process. Following are our steps for developing an effective MCS.
Step 1: When to start? Conservatively, we suggest that if the myopia is already greater than 1.00D before the age of 10 to 12 years, and if the progression exceeds the average of 0.50D/year (Zhao et al, 2002), an MCS should be initiated (optical, pharmacological, or both) (see Step 1 figure). If these conditions are not met (younger, lower myope), but significant genetic risk factors are present, an MCS should also be strongly considered. A more proactive approach would suggest that every child should be managed with an MCS as soon as myopia is clinically visible, but here it comes down to each individual practitioner’s professional judgment.
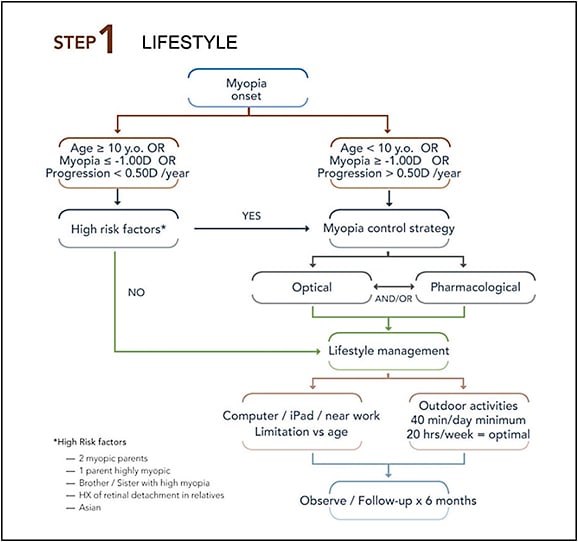
While not often discussed, environmental conditions should be assessed for every child, and both the patients and their parents should be given instructions as part of the MCS. Recommendations include increased outdoor activities (Jin et al, 2015) for a minimum of 45 minutes/day, reduced exposure to technology at near distance (Li et al, 2015), strategies to limit and/or improve convergence (Bayramlar et al, 1999), and decreased distance of near work (Schmid and Strang, 2015). This is especially true if optical or physiological indices are found that are predictive of myopic onset. Young females should be managed more aggressively compared to males, considering that myopia appears sooner for them and evolves more rapidly.
Step 2: Which method to select? Once the decision to implement an MCS is made, choosing the correct method is the next step (see Step 2 figure). The key elements in determining this are the pupil diameter and, not far behind in importance, the amount of the baseline myopia measured under cycloplegia.
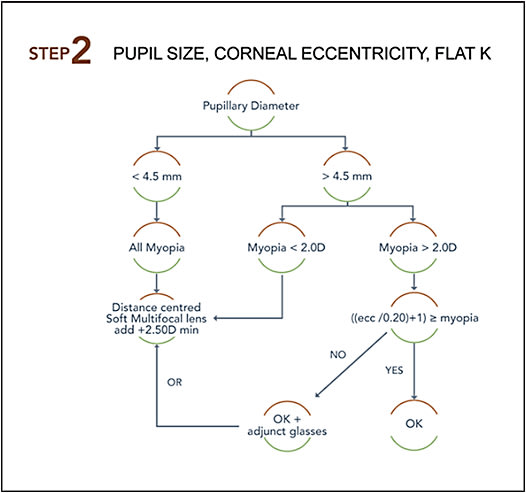
A large pupil facilitates a positive outcome with an OK MCS (Chen et al, 2012). This is a logical finding if we take into account the theory proposed by Smith (2013) that peripheral defocus drives axial length growth, in part, and that an imposed peripheral myopic defocus can slow myopia progression.
An analysis based on more than 200 OK cases (Université de Montréal internal data) showed that the central area flattened by OK lenses (optical zone) varies from 2.8mm to 4.5mm in diameter (average 3.5mm), depending on the design and the base curve radius of the commercial lenses used. This means that the “treatment” zone that generates myopic defocus begins outside of this area. To be effective, most of this treatment zone (1.5mm to 2.0mm) must lie within the pupil area. If located outside of it, then the peripheral refraction will most likely not be influenced, except marginally by peripheral rays entering the pupil by the side. This is why pupil diameter is the first element to consider. Simply stated, if the pupil is not large enough (3.5mm + 1.5mm = 5.0mm), OK won’t be effective in controlling myopia with current commercial designs.
Moreover, in such cases, not only is the myopia progression not reduced, but on the contrary, axial length evolves more compared to single-vision spectacles—0.75mm versus 0.47mm, respectively, within 24 months (Chen et al, 2012). This is due to the fact that a flatter cornea induces more hyperopic defocus at the small pupil’s margin, leading to a myopia increase after a few weeks or months of this increased stimulation. In these instances, the worst-case scenario occurs when practitioners decide to flatten the cornea even more, refitting a flatter lens because of the increased myopia. Then a vicious circle begins in which central flattening pushes myopia even further, and so on.
It is possible to customize OK designs to generate more myopic defocus near the visual axis or to increase the myopia correction beyond 4.00D; however, distance vision may be compromised. The pupil is a dynamic physiological factor; its size varies during the day, driven by lighting and visual tasks, but is not influenced by refractive error (Orr et al, 2015). The MCS should address such variations to make sure that the methods applied are fully effective. This means selecting a method that allows the myopic defocus to lie in the pupil area for most of the visual tasks accomplished during the day.
Once the pupil width is validated, the amount of myopia will influence the selection of the optimal MCS. When fitted with OK lenses, subjects who had a larger magnitude of relative corneal peripheral power change progressed less compared to those who had smaller variations (Zhong et al, 2014). Upon reviewing our results, we determined that the “add” power generated is at least twice the amount of the myopic correction. For example, an OK lens that induces a 3.00D change in the central refraction will generate a 6.00D peripheral power change, or minus defocus. By analysis (Université de Montréal cases), the most stable cases were achieved when an average defocus of at least 4.50D was generated.
To evaluate the potential amount of myopia correction induced by OK, the following equation can be used: potential correction = (maximal corneal eccentricity / 0.20) +1). In cases of limbus-to-limbus astigmatism, in which e values differ greatly between the two principal meridians, this calculation should be performed for each meridian, and dual-axis OK designs must be favored.
If the potential correction is equal to or greater than the amount of myopia, then OK can be considered. Knowing that eccentricity is rarely greater than 0.70, the maximal OK effect will be limited to 4.50D. At the other end, correcting a myopic refractive error of –2.00D will generate only 4.00D of myopic defocus in the periphery, which may be too limited to be effective. If the potential correction is inferior to the amount of myopia, this does not mean that OK can’t be considered—but there will remain a residual refractive error that must be compensated for with single-vision lenses. In fact, partial correction with OK was highly effective on higher myopes (Charm and Cho, 2013).
Even though some OK designs can correct a higher amount of myopia than –4.00D, we’ve found that targeting a correction greater than 4.00D markedly increases the risk of significant central staining that may evolve into corneal erosion and scarring. Moreover, the higher level of correction also increases the risk of a decentered lens/treatment zone. These cases regress more rapidly during the day, which can be annoying for patients.
Because myopia control involves treating children, and we do not want to expose them to such adverse effects, we limit ourselves to 4.00D of OK correction and then rely on glasses for the residual refractive error. The important factor is that the molded cornea is generating enough myopic defocus to be effective in controlling myopia evolution.
If the corneal eccentricity is too low, the potential myopia correction will be limited, and insufficient peripheral defocus will be generated. Other methods will have to be considered in such cases. As a general consensus, any myopia under –2.00D fitted with OK lenses does not generate consistently sufficient peripheral defocus to greatly influence myopia progression when compared to other options. For lower myopes, other strategies should be favored.
One such strategy is soft multifocal lenses. To be an effective option, these lenses must be designed as center-distance multifocal lenses. Some commercially available lenses can be used (mostly off-label). However, most of the commercially available disposable and frequent replacement soft multifocals are manufactured with the opposite design (center-near aspheric).
For the addition power, a +2.00D center-distance lens does not always generate sufficient peripheral defocus in all quadrants, whereas a higher add (+2.50D and above) seems to meet this criterion (Berntsen and Kramer, 2013). Lower add values do not change peripheral defocus and cannot be considered as part of an MCS (Lopes-Ferreira et al, 2011).
Relying on higher add power with distance-center contact lenses can impact distance vision, especially under reduced lighting and contrast (Sanders et al, 2008). This can influence the selection of an MCS. It would be counterproductive to over-minus patients who are fitted to control myopia progression, as it would not be appropriate to leave a young patient with constantly blurred visual acuity at distance. In fact, the latter would be equivalent to under-correcting myopia, which can lead to myopia progression (Smith and Walline, 2015). When considering soft multifocal lenses, practitioners must balance the high add power needed with its impact on distance vision.
Step 3: Perform a baseline evaluation. A practitioner who wants to implement an MCS should rely on valid and extensive baseline data to be able to track the evolution of the refractive error over time. The following testing should be part of any MCS baseline assessment:
• Oriented case history, including past modes of correction, progression rate, clinical signs and symptoms, lifestyle habits, school performance, general health, contributing/risk factors, genetic and ocular background, etc.
• Uncorrected visual acuity (UCVA) and best-corrected visual acuity (BCVA) at distance, near, high contrast, and low contrast
• Pupil diameter measurement under photopic and mesopic conditions
• Manifest refraction (distance, near)
• Binocular vision assessment (distance, near)
• Cycloplegic auto-refraction
• Topographic mapping
• Axial length measurement
• Ocular health evaluation (slit lamp examination, fundus examination)
Step 4: Schedule close follow-up visits. Follow-up visits are crucial to monitor the evolution of a case and to adjust the MCS accordingly. If OK is selected, the first follow-up visit should be performed after the first overnight wear, then seven to 10 days after the initial wear. At this visit, topographic maps, visual acuity testing, and ocular health must be assessed. At every visit, patients must be asked about compliance and provided with recommendations to maintain safe wear. If everything goes according to plan, the next visit will be at three months, then every six months.
In general, an MCS using OK lenses is expected to slow myopia progression by 45% (Sun et al, 2015). This is comparable to the results obtained with soft multifocal lenses (slightly less than 50% reduction [Walline, 2016]). Recent data are promising, as authors are reporting a 70% myopia progression decrease using a combination of distance-center bifocal contact lenses and bifocal glasses to neutralize the associated phoria (Aller et al, 2016).
The best indicator of myopia progression is the variation of the axial length. It should be evaluated periodically, in the context of cycloplegic refraction, to determine whether myopia progression occurs.
The Step 3 figure describes troubleshooting options relative to OK lens wear. If the results do not meet treatment goals, other options should be considered. Patients can be switched to multifocal soft lenses or be prescribed additional pharmacological treatment, such as atropine 0.01% (Chia et al, 2016). There are some gaps in the understanding of the favorable outcome obtained with anti-muscarinic drugs. For example, the application of atropine 0.01% stopped myopia evolution, but axial length still progressed, which raises questions about a potential rebound effect occurring. We would suggest that atropine may play a role with the crystalline lens, helping to balance axial length growth with the refractive power of the eye, which could limit this rebound effect.
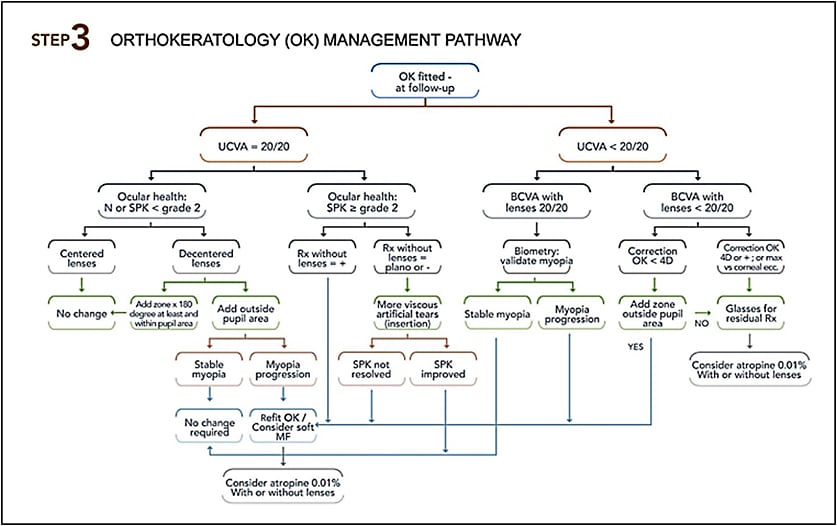
Similar to OK lenses, follow-up visits for soft multifocal lenses must be performed seven to 10 days after the lens delivery, then at one month, three months, and every six months thereafter. Table 1 lists the testing necessary to assess myopia evolution over the course of a year. The Step 4 figure provides trouble-shooting for a multifocal soft lens MCS.
Baseline/annual | 24 hours | 7 to 10 days | 30 days | 3 months | 6 months | |
---|---|---|---|---|---|---|
Oriented case history | X | X | X | X | X | X |
UCVA/BCVA | D, N - HC, LC | D - HC | D - HC | D, N - HC | D, N - HC | D, N - HC |
Pupil diameter | X | |||||
Manifest refraction | D, N | D, N | D, N | |||
Binocular vision | X | |||||
Cycloplegic auto-refraction | X | |||||
Topographic maps | X | X | X | X | X | |
Axial length | X | X | X | |||
Ocular health | X | X | X | X | X | X |
X = Testing performed | ||||||
D = Distance, N = Near, HC = High contrast, LC = Low contrast |
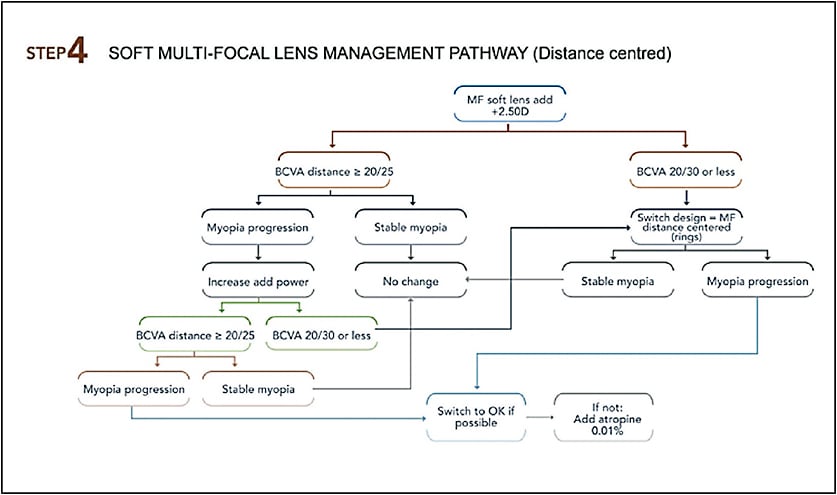
Conclusion
Eyecare practitioners should now perceive myopia as an important risk factor leading to visual impairment and potential blindness. Myopia control strategies should be initiated with every myope as soon as possible—or, at the very least, as soon as myopia is observed to be increasing at a rate that is higher than expected. The goal is to keep the amount of myopia as low as possible to alleviate ocular pathology.
Many elements should be considered before selecting the optimal MCS. Pupil diameter is a key factor, followed by the baseline level of myopia and corneal eccentricity. Lifestyle change is as important as optical management, and it needs to occur to optimize MCS results.
An MCS can include the use of OK lenses, soft distance-center multifocal lenses, and medication. None of these options is successful with every patient, and selecting the optimal option needs to occur on a patient-to-patient basis.
For example, OK is preferred in patients who have larger pupils, higher myopia, and who are progressing at a faster rate. In other instances, OK lenses may increase myopia instead of reducing its rate of progression. Soft distance-center multifocal lenses are the go-to solution for smaller pupils, emergent or lower myopia, or low corneal eccentricity. However, a positive outcome in this case really depends on an optimal design, which is still to be defined.
Medication can be used as adjunct therapy or as a stand-alone strategy, but it should not be considered the first-line option. On that point, for the time being, more long-term studies are needed, although anti-muscarinic drugs are showing very favorable results.
An MCS provides a tremendous opportunity for eyecare practitioners to help their patients and to apply their expertise. It’s time for all of us to pay attention to the rapid evolution in the field and to begin talking about myopia control with every young patient entering the clinic. CLS
For references, please visit www.clspectrum.com/references and click on document #244.
![]() | Dr. Michaud is a full professor and chief of the contact lens department at École d’optométrie de l’Université de Montréal. He is also a consultant or advisor to Blanchard and has received educational funding from Allergan; research funding from Blanchard, Alcon, and Johnson & Johnson Vision Care; travel funding from Genzyme Canada and SynergEyes; and lecture or authorship honoraria from Blanchard, CooperVision, B+L, and Allergan. |
![]() | Dr. Simard is in private practice at Clinique d’Optométrie Bélanger and is a clinical instructor and lecturer at Université de Montréal. |
![]() | Dr. Marcotte-Collard is in private practice at Clinique d’optométrie Bélanger and is a clinical instructor in the contact lens clinic at Université de Montréal. He completed a contact lenses and dry eye residency under the supervision of Drs. Langis Michaud and Etty Bitton. He is now a master’s candidate at Université de Montréal. |