Orthokeratology (ortho-k) is a well-established process by which the corneal surface is reshaped to correct refractive ametropia through the use of specific contact lens designs. Ortho-k uses reverse geometry lenses that are specially designed to have very low clearance over the pupil. These designs are comprised of different zones that either provide treatment or stabilize the lens.
THE ORTHO-K LENS DESIGN AND HOW IT WORKS
Even though ortho-k lenses exhibit apparent central bearing with their characteristic “bull’s-eye” fluorescein pattern, a thin tear lens remains at the apex; between 5 and 15 microns of clearance is usually acceptable. This area of apparent bearing corresponds to the optic zone of the lens, commonly around 6mm in diameter. Just outside of the optic zone is the area that generates the tear reservoir from the reverse curve (RC). The RC is usually about 3.00D steeper compared to the back optic zone radius over an area 0.50mm to 1.00mm in width.
Stability and centration are critical to success when fitting ortho-k lenses. Peripheral curves play an integral role in this stabilization and also serve in closing off the lens fluid system. Typical GP lenses have flatter peripheral curves to achieve edge lift and allow tear exchange under the lenses. Ortho-k lenses are designed to be in close alignment with the peripheral cornea just outside of the optic zone, giving this area the appropriate name of the alignment zone, which is typically about 0.70mm to 1.00mm in width. The outermost zone is the peripheral curve system; these are the flattest curves, about 0.50mm wide, which provide edge lift for the lens.1 Figure 1 shows a general ortho-k lens design.
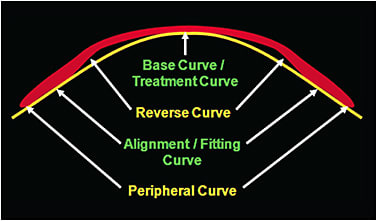
One theory regarding how this design works is that it creates fluid forces beneath the lens to move corneal tissue. Centrally, a positive pressure force is created from the low clearance, much like capillary action, which pushes tissue out away from the apex. Conversely, the fluid reservoir under the RC generates a negative fluid force that pulls corneal tissue into it. The combined efforts of these two forces result in central flattening, which clinicians use to correct myopia.
However, the back optic zone radius (or base curve) and the RC are not individually responsible for these forces, but rather it is the combination of the two that creates this effect. Together, the radii (or angles) and the diameters of these zones create the sagittal height (sag) of this central system in which all of the biomechanical forces of the procedure occur.
For the treatment to be most effective, the design must account for corneal shape factors such as curvature, astigmatism, and eccentricity. Additionally, topographical elevation data should be acquired and taken into consideration so that the lens has a similar sag to the cornea over a given chord length.
SPHERICAL ORTHO-K DESIGNS AND ASTIGMATISM
Perhaps the most common issue encountered in ortho-k fitting is lens decentration, often perceived to be induced by corneal toricity (Figure 2). This decentration can result in induced irregular astigmatism and subsequent decreased visual acuity, decreased image quality, and glare.2,3 Traditionally, spherical ortho-k lens designs have been contraindicated beyond 1.50D of with-the-rule refractive astigmatism.2
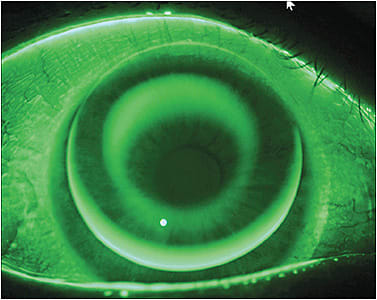
There has even been a question as to whether ortho-k lenses can induce astigmatism on clinically spherical corneas. However, research suggests that ortho-k does not cause significant refractive astigmatism in non-astigmatic patients; unanticipated subjective refractive changes may, however, result from an increase in higher-order aberrations.4
In some reports, spherical reverse geometry designs have been found to reduce up to 60% of initial with-the-rule astigmatism.3-5 However, in many of these cases, the astigmatism was isolated to the central apical region of the cornea. A majority of the treatment occurred within the central 2.00mm chord, and there was little reduction of astigmatism beyond 4.00mm from the corneal apex.6 It is postulated that greater amounts of astigmatism or limbus-to-limbus astigmatism inhibits fluid pressure forces by preventing 360º seal-off in the area of the alignment curve. Failure to achieve this seal-off allows fluid to escape along the steep meridian, thereby diminishing the negative fluid force produced by the tear reservoir (Figure 3).
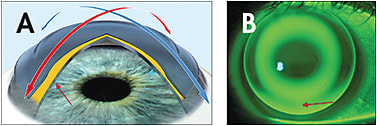
A possible method of predicting and managing this complication is by looking at topographical elevation maps. This elevation data may guide practitioners to a design that fits each corneal meridian independently, so that full treatment can be obtained in the more myopic axis.3
INTERPRETING AND ANALYZING CORNEAL TOPOGRAPHY
It is widely accepted that corneal topography is a key, if not the most important, element in monitoring corneal reshaping patients. Topography records a baseline that allows for monitoring of corneal change over time with the utilization of difference, or subtractive, maps. Axial, or sagittal, maps show the radius of the corneal surface perpendicular to the reflected mires, or the Placido disc in most systems. This is used to analyze the optical effects of corneal shape.7 These maps are typically displayed in diopters based on local radius of curvature; they should not be interpreted as refractive power, but rather as curvature maps (Figure 4). These maps provide an estimate of surface refractive power only at the corneal apex. Sagittal maps are typically used in contact lens fitting because they provide a general sense of how flat or steep a cornea is shaped.8
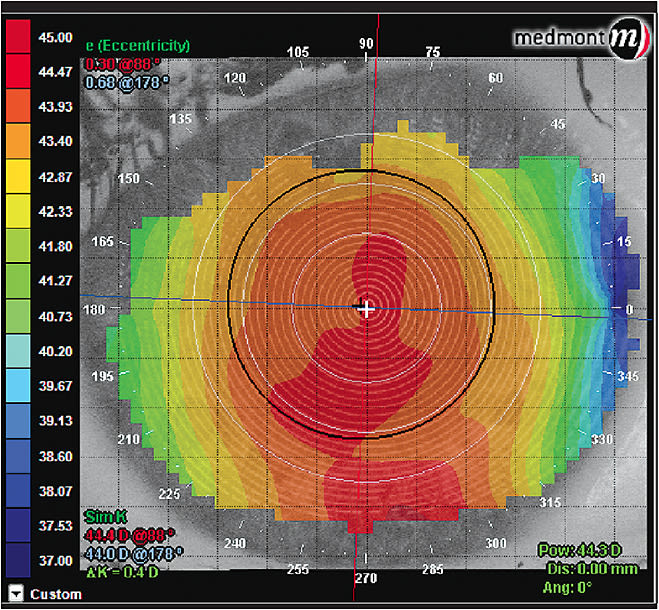
Tangential, or continuous, maps show the radius of curvature of the corneal surface that is contained within the mires. These maps provide better representation of corneal shape because they are not based on the optic axis.7 Tangential maps are more sensitive for surface shape changes because the instantaneous radius increases from the center to the periphery more quickly for an ellipsoid compared to axial radius. This is useful for detecting keratoconus and other corneal disease and for showing optical effects of astigmatism (Figure 5).8
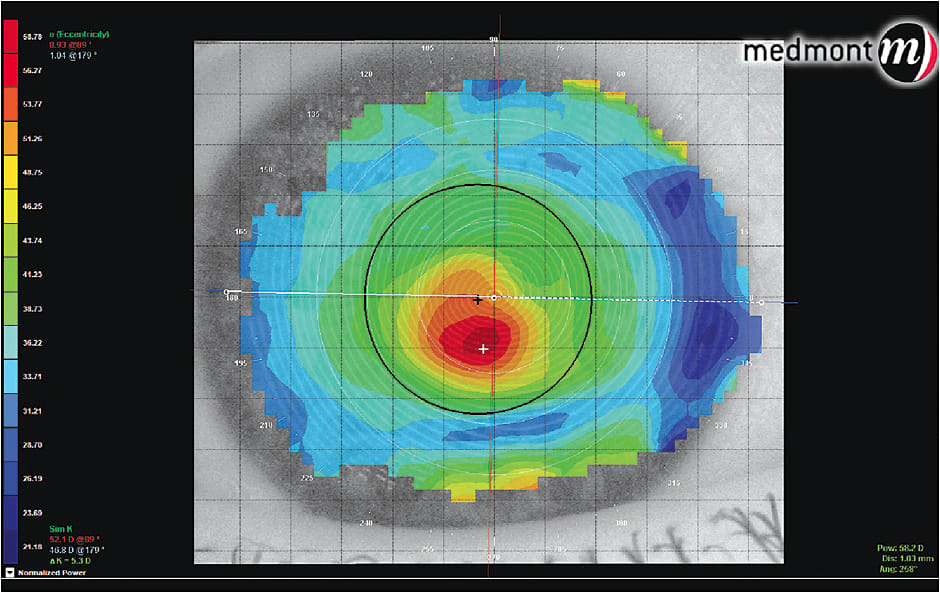
Elevation maps show the corneal sagittal height in microns from a flat plane fixed in place. This data is useful for evaluating true corneal shape clinically. However, elevation maps are not good at showing subtle variation.7 This can be improved by applying a “best-fit sphere,” which greatly impacts the accuracy of the map, depending on position and radius.9 This reference sphere allows subtle variations, tens of microns in this case, to be displayed and not lost in the relatively large sag of the rest of the cornea. These small-micron differences can be significant optically and with regard to fitting contact lenses. This information on the subtle variations of corneal shape can also be useful in evaluating treatment outcomes of refractive surgery.8
Topographical difference maps usually show three sections. The top left is the pre-treatment data, the lower left is the post-treatment data, and the right map shows the point-to-point difference between the two. Axial difference maps show the change of corneal curvature in the treatment zone, typically how much the cornea has been flattened. Although this can provide some sense of centration, this can be better assessed with tangential difference maps. On a normalized scale, these maps have a red ring that indicates where the corneal epithelium has been redistributed, or the area in which the cornea has been steepened. This red ring should be centered over the pupil to ensure that the optic zone is optimally positioned.7 The maps are compared side by side in Figure 6.
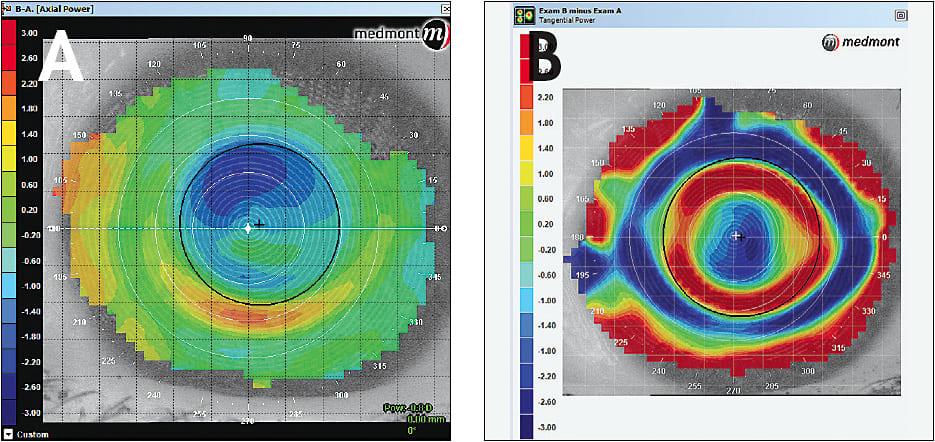
EFFECT OF CORNEAL ASTIGMATISM
As corneal astigmatism increases or its area extends beyond the central apex of the cornea, it can become more difficult to achieve optimal treatment with a spherical ortho-k lens. This is largely due to the effect of corneal elevation differences farther out on the corneal periphery. Corneas that have limbus-to-limbus astigmatism will likely have a disparity in elevation between the horizontal and vertical meridians at this distance from the apex (Figure 7).
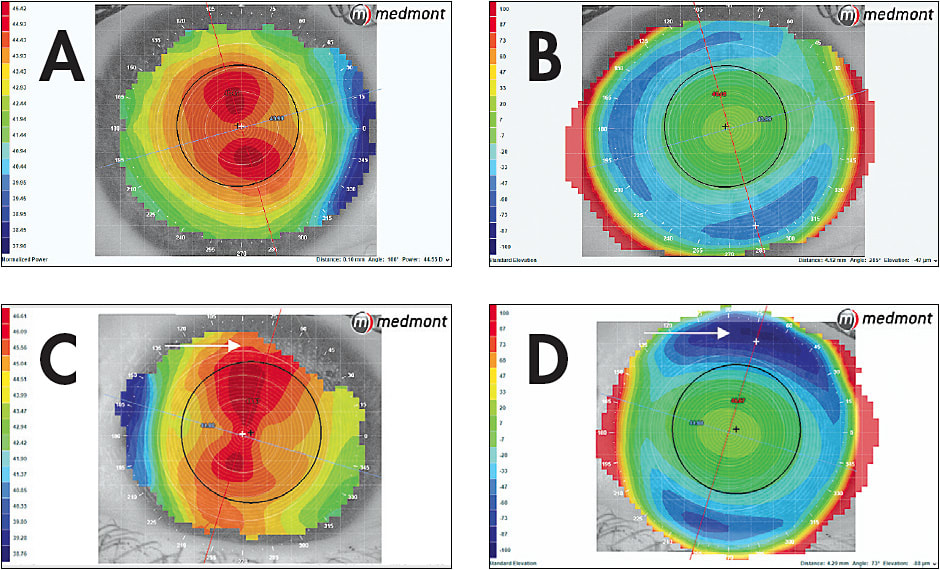
Some manufacturers manage these fitting needs by incorporating meridian-specific reverse curves and alignment curves. The overall effect is a difference in the sag of the tear reservoir. Figure 8 shows the topographical results of these meridian-specific designs.
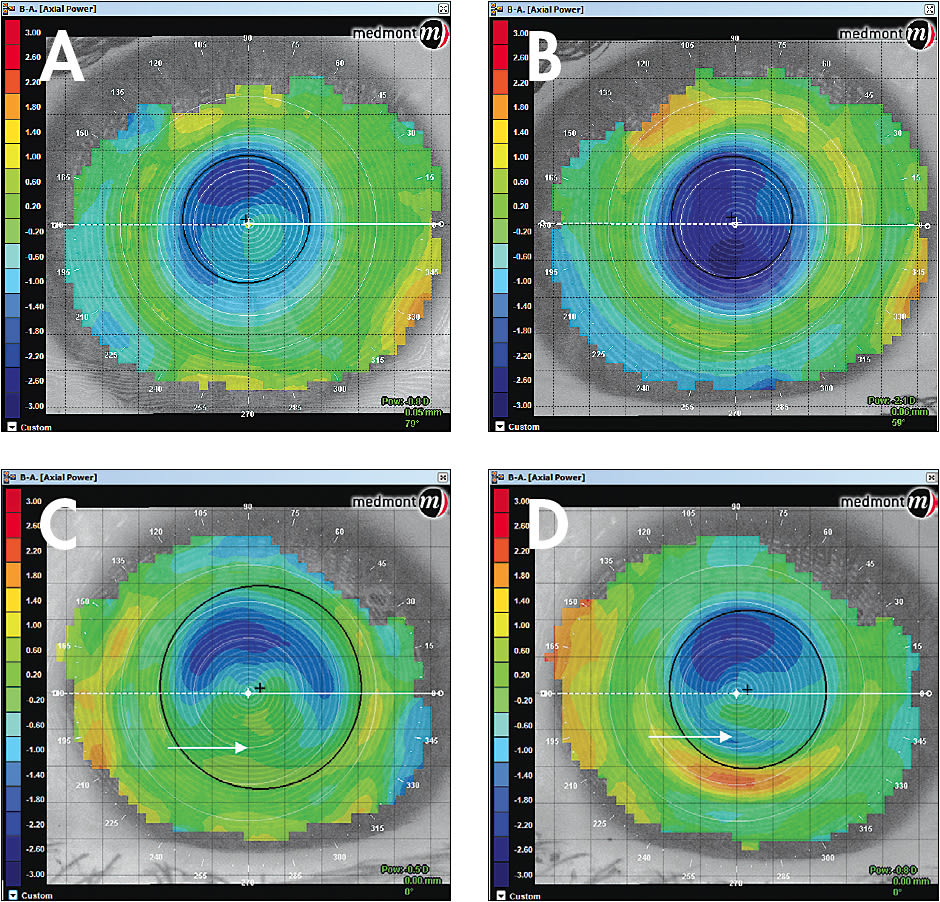
Corneal astigmatism in itself does not directly correlate to a significance in corneal elevation differences. Morgan et al looked at the relationship between corneal astigmatism and elevation difference in a population of people 22 to 40 years old.10 The study examined 121 eyes from 62 subjects retrospectively, assessing corneal curvature (sim K) and elevation differences (taken at the 8mm chord) along the two principal corneal meridians (Figure 9).
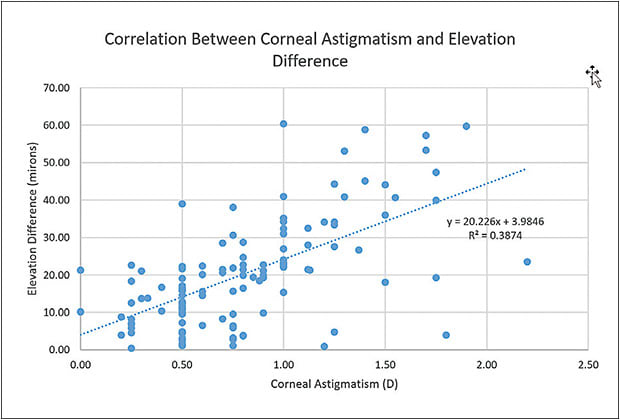
These data mean that a patient can have a spherical cornea as well as a significant elevation difference. For the study, a spherical Paragon CRT (Paragon Vision Sciences) lens was placed on the eye. The lenses had an optic zone diameter of 6.00mm and a 1.00mm wide reverse curve; therefore, the alignment curve began at about 4.00mm from the center of the lens. The alignment curve comes in contact with the higher elevation points, usually the horizontal/flat meridian, and lifts off in the axis of lower elevation, usually the vertical/steep meridian. This lift can result in decentration and decreased treatment effect with a spherical lens (Figure 10).
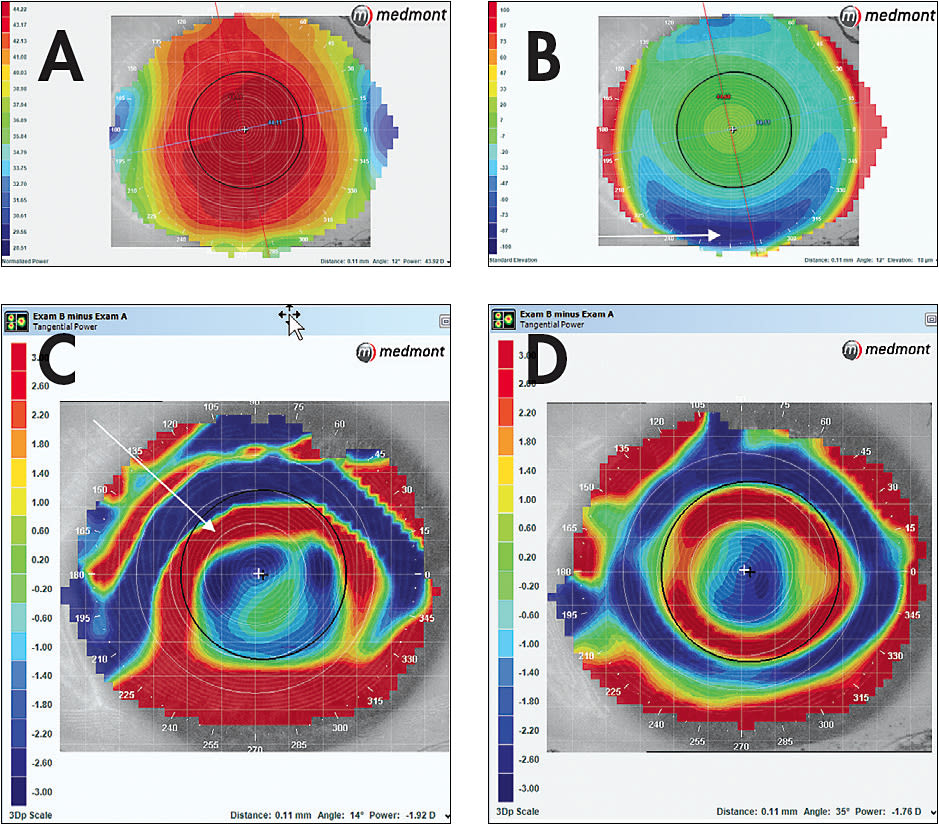
A smaller sample of subjects was isolated to see the comparison of a spherical lens versus a meridian-specific lens on spherical and toric corneas with or without spectacle astigmatism, resulting in four combinations of eyes versus astigmatism. Figures 6 and 8 show the effect of these lenses.
CONCLUSION
Elevation data should be considered when fitting any ortho-k patient, whether spherical or astigmatic. Meridian-specific lens designs can improve centration as well as treatment efficacy in both types of patients.
The question then becomes when is the elevation difference significant and at what chord length should it be measured? This question is still being studied, but some fitting guides recommend implementing a meridian-specific design at as little as 25 microns of elevation difference. It could be argued that the chord length measured should be 9mm rather than 8mm for designs that have 6mm optic zones, as 9mm would be where the lens actually lands. The next decision is whether to have meridian specificity in the reverse curve, alignment curve, or both. More research needs to be performed to answer these and other questions. CLS
REFERENCES
- Mountford J. Design variables and fitting philosophies of reverse geometry lenses. In Mountford J, Ruston D, Dave T: Orthokeratology Principles and Practice. London. Elsevier, 2004:69-107.
- Chen CC, Cheung SW, Cho P. Toric orthokeratology for highly astigmatic children. Optom Vis Sci. 2012 Jun;89:849-855.
- Pauné J, Cardona G, Quevedo L. Toric double reservoir contact lens in orthokeratology for astigmatism. Eye Contact Lens. 2012 Jul;38:245-251.
- Cheung SW, Cho P, Chan B. Astigmatic changes in orthokeratology. Optom Vis Sci. 2009 Dec;86:1352-1358.
- Mountford J. An analysis of the changes in corneal shape and refractive error induced by accelerated orthokeratology. Int Contact Lens Clin. 1997 Jul-Aug;24:128-144.
- Patterson TC. Orthokeratology: changes to the corneal curvature and the effect on refractive power due to the sagittal length change. J Am Optom Assoc. 1975 Jul;46:714-729.
- Dave T. Corneal topography and its measurement. In Mountford J, Ruston D, Dave T: Orthokeratology Principles and Practice. London. Elsevier, 2004:17-49.
- Salmon TO, Horner DG. Comparison of elevation, curvature, and power descriptors for corneal topographic mapping. Optom Vis Sci. 1995 Nov;72:800-808.
- Gatinel D, Malet J, Hoang-Xuan T, Azar DT. Corneal elevation topography: best fit sphere, elevation, asphericity, toricity, and clinical implications. Cornea. 2011 May;30:508-515.
- Morgan B, Gidosh N, Rosen C, Norman C. I’ll have a corneal reshaping lens please...with a twist. Clinical Science Poster presented at the Global Specialty Lens Symposium, Las Vegas, January 2016.