The science and art of contact lens fitting can broadly be divided into two different but not necessarily mutually exclusive approaches—diagnostic and empirical. Diagnostic and empirical fitting of contact lenses have represented the primary methodical techniques to successfully achieve the desired triad when utilizing contact lenses: good visual acuity, good subjective comfort, and good ocular physiology.
THE DIAGNOSTIC METHOD
Each approach has pros and cons. And, when approaching GP lens fitting, most practitioners historically have relied more heavily on diagnostic fitting, at least in part due to empirical fitting previously leading to only 40% first-lens success.1 Diagnostic fitting might also be a default approach for many eyecare professionals (ECPs) due to the availability of diagnostic fitting sets and to a certain comfort zone for them (i.e., that’s the way it’s always been done).
Certainly, one of the great advantages that diagnostic fitting provides is that many fitting hurdles can be addressed before any patient-specific lens is designed and manufactured. The relationship of the back surface of the lens with the ocular surface can be assessed and modified through subsequent diagnostic lenses until the most ideal relationship is achieved. The potential for visual acuity, along with perfecting the visual performance by optical power manipulations of the trial lenses and over-refractions, can also be assessed and idealized during diagnostic fitting.
Obtaining this information prior to committing to manufacturing a customized patient-specific lens is clearly advantageous for both patients and practitioners. This provides patients with a glimpse into the proper expectations of the lens performance once a proper lens is manufactured for them. It also provides sufficient information to almost guarantee that the first lens manufactured should provide good vision, good comfort, and good physiology and, therefore, should be possible to dispense. Also, once a laboratory has had to manufacture a custom lens for a patient, there is an increased financial burden that must be borne by the patient, the practitioner, the laboratory, or some combination of all three participants. Obtaining such necessary information prior to committing to proceed—by involving the manufacturing laboratory—means that diagnostic fitting can lessen this increased financial burden. This is especially true if the final lens ultimately fails to meet the patient’s and/or practitioner’s expectations.
However, performing all of the fitting through this diagnostic process is not without a negative side. Diagnostic lens fitting requires fitting sets that are appropriate for the end design being targeted. For example, trial fitting with a set of single-vision spherical lenses will provide very little value when the desired design is a translating bifocal that includes prism. As there can be a wide variety of lens categories typically prescribed in a practice, several fitting sets would be required to gain true value from trial lens fitting. Because many labs may offer designs for categories such as multifocal lenses, scleral lenses, orthokeratology, etc., the designs have their own unique proprietary fitting sets; this means that the trial lens fitting typically needs to stay within that particular branded product line.
It would not be uncommon to perform a trial fitting with a specific branded design, attempting several lenses and varying the parameters to continually improve the fit, only to find that some limitations inherent to that design ultimately prevent a successful or ideal outcome. This would then necessitate additional trial lens fitting with a different branded design. This costs office time as well as the patient’s time. Fitting exclusively through diagnostic fitting sets also limits ECPs to prescribing only from this subset of available designs. In a given category of lenses, such as orthokeratology, an ECP can be successful only with patients who are within the guidelines of the specific orthokeratology diagnostic sets that are available in his or her practice. This leaves many patients outside of those guidelines who could still be successfully managed with orthokeratology—just not by that practitioner.
In addition, as the rotational asymmetry of lens designs has continued to increase, the impracticality of having a representative diagnostic set that is robust enough to contain the needed nuanced parameters has become obvious. It has become less practical to perform diagnostic lens fitting because diagnostic lens sets are becoming woefully inadequate at representing the parameters required for a lens that ultimately will be successfully fit.
To properly maintain fitting sets, their parameters need to be continuously verified. Additionally, proper disinfection protocols need to be implemented between uses. Recommendation guidelines for these protocols (based on the 2018 International Organization for Standardization [ISO]) were revised and released in early 2020.2 The disinfection protocols are extensive, which adds a cost burden and affects office efficiency. Diagnostic fitting—possibly requiring many lenses, during which the patient likely has poor vision and after which the patient doesn’t even have the opportunity to leave with the lenses—is not a positive patient experience. Additionally, if many lenses were used during this process, a diagnostic fitting can represent a trying physical and possibly emotional experience for patients, a great expenditure of their time, no improvement in vision, and sore irritated eyes.
THE EMPIRICAL METHOD
Alternately, employing an entirely empirical fitting process is a very different experience for patients. At its roots, this process consists of the collection and interpretation of data, the application of knowledge and experience to guide the design of the contact lens, and the subsequent manufacture of a custom contact lens product specific for that patient. The first lens that patients experience on their eye is one that has been specifically tailored to their unique ocular physical needs and to their refractive requirements.
From a patient experience perspective, this can be incredibly positive and even exciting, especially in the common scenario in which the eye does not correct well with spectacles due to a corneal irregularity. In this scenario, patients have been enduring poor-quality vision that has not been possible to correct properly with spectacles, perhaps for many years. A contact lens that not only corrects ametropia but also has the ability to neutralize a large portion of the aberrations that result from the corneal irregularity can be a revolutionary visual experience; it can bring an almost overwhelming amount of positive emotion along with a sudden revelation into bright and clear, well-corrected vision. This is also efficient and positive for practitioners, as it saves chair time as compared with diagnostic fitting.3
Historically, the initial data collected before empirically designing lenses would have included many items, starting with the spectacle refraction and the best-corrected acuity. If contact lenses were already being worn, ECPs would evaluate the acuity with the contact lenses, any over-refraction that might have improved the vision, and the fit of the lenses. The lens specifications such as diameter, base curve, back optic zone, lens power, and widths of the peripheral curves would be measured or obtained from a written prescription. If a specific branded design was used, that would be useful information. Patients’ horizontal visible iris diameter and the position of the upper and lower lids as well as their relationship to the globe would be determined. The pupil size in photopic and scotopic conditions would be evaluated, and manual keratometry would be performed. If topography was available, sim Ks, overall distribution of the curves, positioning and extent of the cylinder, eccentricity values and how they vary from quadrant to quadrant, and overall corneal asymmetry would be assessed. A qualitative and quantitative evaluation of the tear layer would be performed including direct observation at the biomicroscope of the tear meniscus volume and composition, tear breakup time, Schirmer’s testing, and vital dye staining. Lid margin, conjunctiva, and iris color would all be evaluated prior to beginning an empirical contact lens fit.
As there appears to be a significant advantage in creating a more positive, efficient approach through empirical fitting, and as the data collected is extensive, one question to consider is: Why has there historically been such a poor first-lens success rate when empirically fitting contact lenses?
Beginning with the data collection, empirical fitting largely consisted of manual keratometry, which provided two points of curvature references at a chord of 3mm. Relying on just two instantaneous curvature points to describe the incredibly complex and varied shapes that corneas can have was probably one of the weakest ways to extrapolate a lens design that would perform properly. For example, the keratometry data collected might indicate a very average curvature of 44.00D/44.50D. However, very high eccentricity values, a high degree of rotational asymmetry, or even a local irregularity would make that cornea anything but average. A cornea that has a diameter of 11.0mm has a much lower sagittal depth compared to a cornea that has a diameter of 12.5mm, yet the keratometry data would not be capable of differentiating these very different shapes. In Figure 1, both corneas have equal and relatively flat keratometry readings of 41.50D/42.00D. The upper cornea has a diameter of 11.2mm, and the lower cornea has a diameter of 12.7mm; therefore, the respective sagittal depths are 2,890µm and 3,410µm, which would require very different lenses.
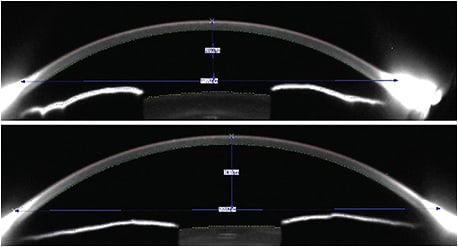
With the strides made in corneal topography acquisition, along with greater general acceptance of corneal topographers in the clinical setting, the two curvature reference points from the manual keratometer have been increased to thousands, tens of thousands, and even hundreds of thousands of reference points to describe the ocular surface. Recreating and modeling the ocular surface (Figure 2) within the computer environment, with curvatures having resolution accuracy finer than 0.10D, have become clinically commonplace.4 Having an increased understanding of the ocular terrain with such exceptional detail has not only benefitted rigid contact lens fitting, it has also improved the clinical insight for soft lens fitting. The rigid lenses, however, have had the greatest improvement benefit, as empirically fit rigid lenses were even preferred to soft toric lenses when patients had the option of choosing.5
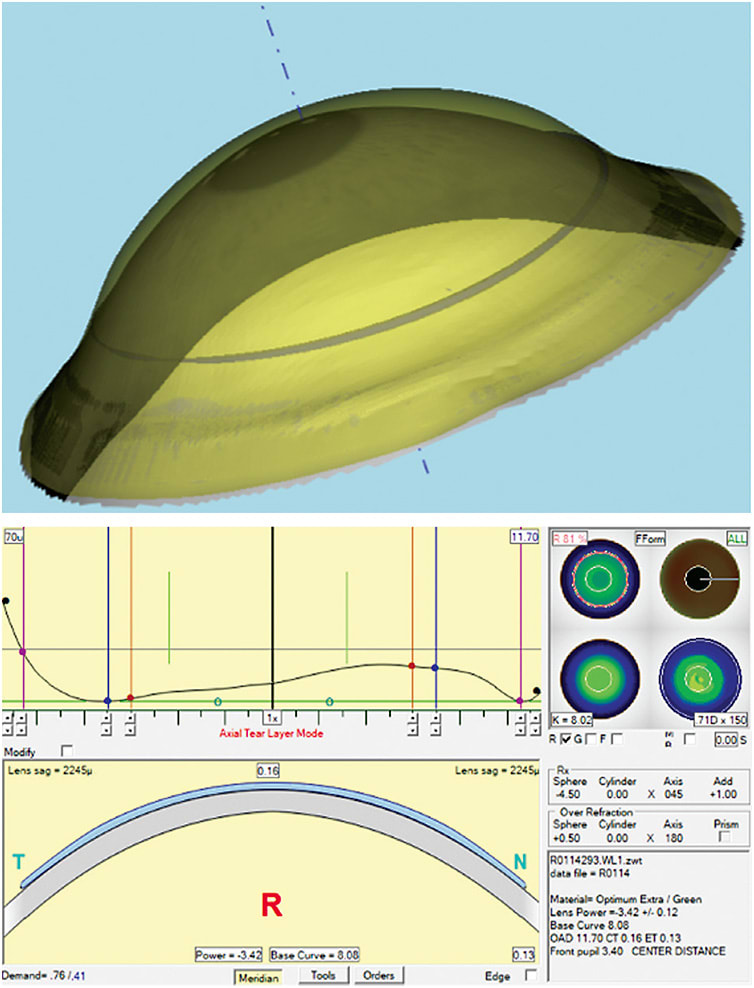
The combination of increased resolution of detail obtained from more modern corneal measuring instruments and the ability to model the patients’ cornea within the computer environment with great accuracy and precision is a monumental leap forward, but this is only part of what is needed to improve the empirical fitting process for rigid lenses. What is also needed to take full advantage of all of this measured data is the ability to translate this information into a complex lens product capable of addressing the underlying asymmetry of the cornea. Finding that the sagittal height of the nasal peripheral cornea is much lower compared with the rest of the peripheral cornea is good information to know but still ultimately leaves the lens as a compromised design if it is incapable of being designed and manufactured to address this asymmetric issue.
Providing improved contact lens approaches to increasing numbers of irregular corneas resulting from disease, trauma, infectious sequelae, and refractive surgery—parallel with the growth of scleral lens use for the above conditions as well as for additional conditions such as ocular surface disease—has forced change for contact lens fitting. In addition to the evolutionary improvements in the diagnostic technology and the growth of corneal topographers in clinical practice, the past few years have produced several scleral mapping topographers. Figure 3 shows some of the displays of these instruments. These topographers provide clinicians with a wealth of data from the center of the cornea to chords as large as 22mm.
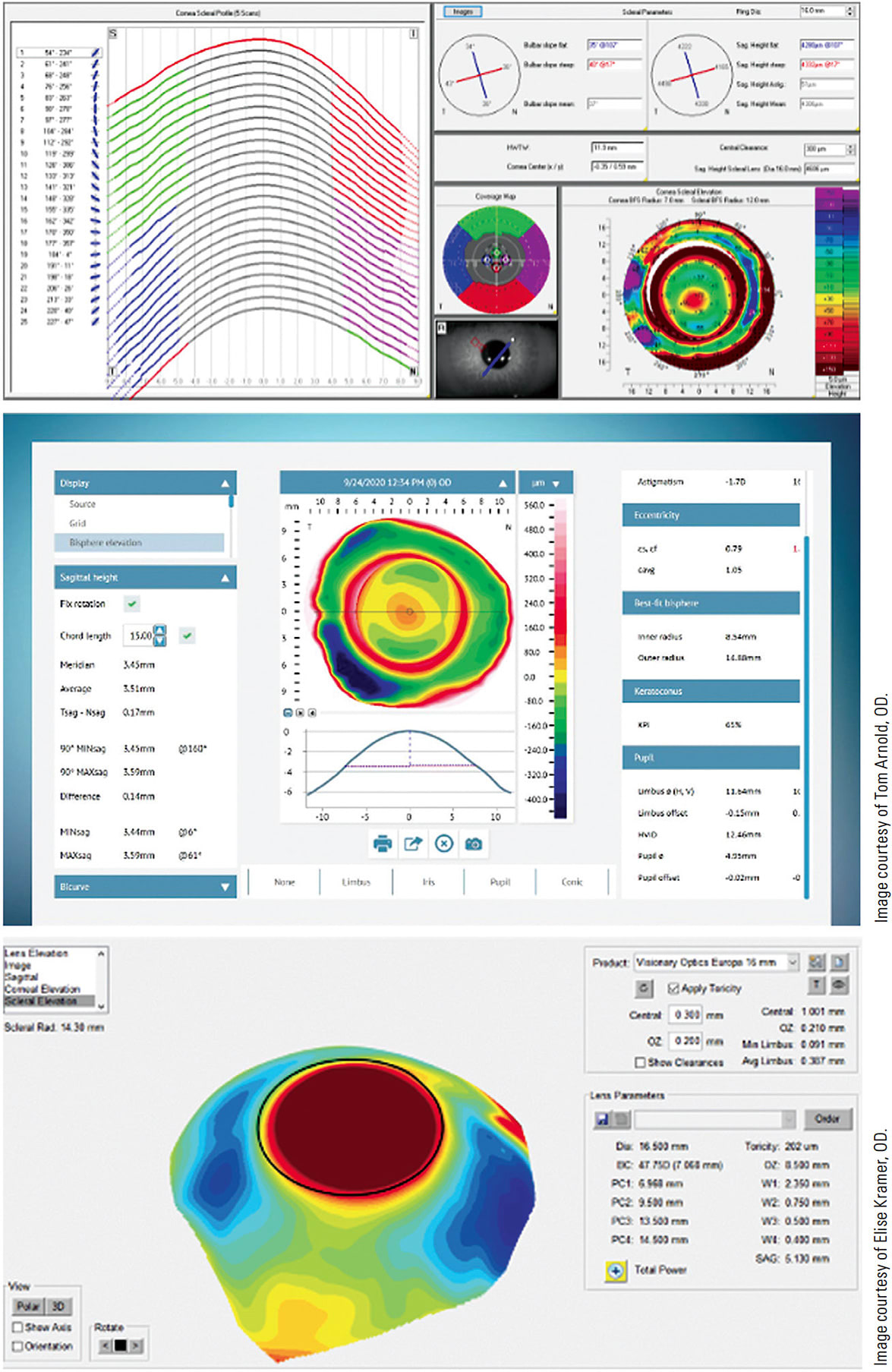
The contact lens products available have continued to evolve, becoming increasingly complex. Virtually non-existent are the simpler rigid designs that started with monocurve lenses and grew into more specified curves that have blended zones. Aspheric curves were added to the peripheries to help better align the lens with the cornea that is flattening peripherally. Rotationally asymmetric rigid lenses in all sizes, ranging from the relatively small corneal lenses to very large scleral lenses, have entered the armamentarium of clinicians. These lenses can better address the physically unique ocular shapes along with the aberrations contained within the complex optics of these tainted visual systems.
TECHNOLOGICAL ADVANCEMENTS
To efficiently manage this increased volume of data that is both collected and manipulated into a lens design, software systems have become an increasingly important part of the empirical fitting process. Nomograms, fitting guides, and over-the-phone consultations with laboratories are somewhat limited in the empirical process when utilizing software to specify thousands of parameters into a lens design (Figure 4). This means that a certain skill level must be mastered by the lens designer when employing the software to design a one-of-a-kind lens based on the unique data collected from that specific eye. This also shifts the responsibility of the design from the laboratory to the practitioner. Branded product lines were married to the diagnostic trial lens approach. The laboratory produced a product line through extensive research and development, real-world trial fitting, and performance assessment. Refinement of the design also included proprietary features that were then manufactured and branded. A representative subset of that specific product line was then created and labeled as a diagnostic fitting set. Practitioners would then utilize the diagnostic fitting set to reach the ideal model for that specific lens design on patients and then specify a patient-specific lens within the boundaries of the branded product. Practitioners utilizing software to design patient-specific lenses, limited only by the manufacturing specifications of the lathe, means that every lens is unique and has not had the benefit of the extensive research and development of the branded products.
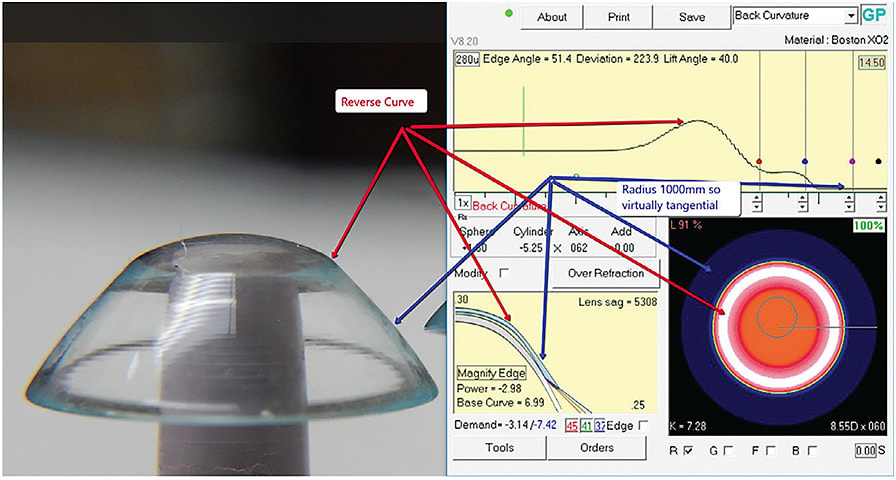
Design software now gives practitioners the control to manipulate thousands of curves while also enabling communication of this volume of information to a computer-numerical-control (CNC) lathe to manufacture the desired design. This opens up the design of the lens to almost limitless possibilities, which means that it is possible for clinicians to define and create a lens capable of the proper relationship for some of the most complex ocular surfaces. Of course, without the safety net of the research, development, and real-world testing of the branded products, the final product designed utilizing the software can also fail to perform acceptably due to inadequate skill on the designer’s part.
Three orthokeratology cases using this empirical software approach were presented in 2004 that demonstrated the breadth of this approach in exceeding what was possible with diagnostic approaches.6 Figure 5 shows the topography of a –9.00D myopic eye with a relatively flat cornea (40.94D @ 004 / 41.72D @ 094) that was reduced to plano with a custom orthokeratology design. This result was achieved with the first lens that was designed empirically, utilizing the topography of the patient to directly guide the design. What was generally thought to be beyond the limits of orthokeratology more than 15 years ago was instead shown to be a limitation of the orthokeratology designs available with diagnostic fitting.
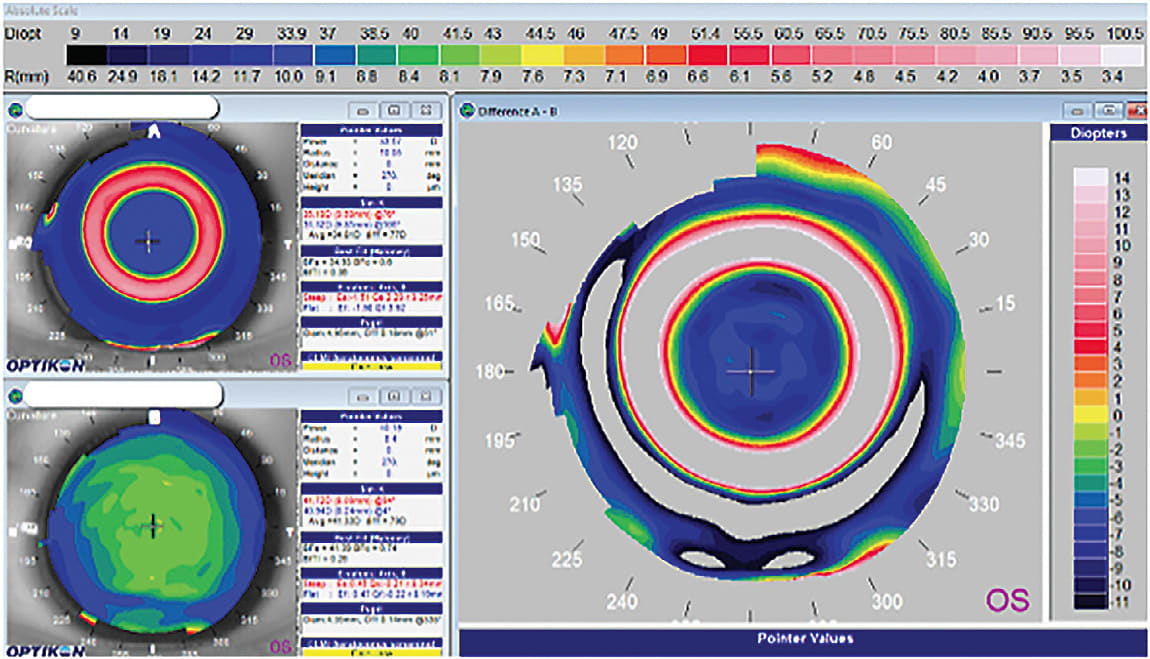
More recently, analogous limitations of scleral lens designs that are fit diagnostically are vanishing with the software approaches for fitting empirically. The scleral shape in approximately two-thirds of eyes has been shown to be asymmetrical.7 This means that symmetrical scleral designs, including lenses that have regular toricity, are, at best, not an optimized fit and, at worst, will fail or potentially cause complications. These more customized lens designs are capable of maximizing patient comfort and vision, while avoiding physiological complications that might arise from less customized designs typically fit through the diagnostic approach.8,9
Empirical fitting is a much more streamlined process; all of the data is collected, the lens is designed and ordered based on the collected data, and the first lens can be very close to a final lens. This is even true when fitting very challenging eyes. As both corneal and especially scleral rigid lenses are typically used when corneal irregularities are present, getting the first empirically designed lens optically perfect can be challenging. For this population, spectacle refractions are often unobtainable or useless when calculating the needed powers of the rigid lens.
There are also instances in which the ocular surface data collected for empirical design can be imperfect. This occurs when the measurement technology encounters difficulties with some of the unique features of irregular eyes. However, untrustworthy data collection continues to be a decreasing problem, as both the hardware and the software used to collect the data continue to improve.
THE WAY THINGS ARE HEADING
Practitioners recently have become more receptive to and are embracing empirical fitting, but diagnostic fitting certainly has not been replaced. There has, however, been a steady—although generally slow—acceptance by clinicians to replace diagnostic fitting with empirical fitting. There does appear to be an external event acting as a catalyst that is disrupting the status quo. The COVID-19 pandemic has caused practitioners to re-evaluate policies, procedures, techniques, and approaches to all facets of patient care. The newer in-office protocols for disinfection of diagnostic contact lenses between uses, combined with the public’s fear of having lenses on their eyes that have been on other people’s eyes, have made clinicians more receptive to change in the contact lens fitting process. There is also the practical issue with diagnostic fitting for true specialty GP lenses that require the purchase and maintenance of multiple fitting sets, each with its own unique approach and respective learning curve. This, in addition to the limitations within the final designs of lenses fit through diagnostic sets, has made the approach of empirical fitting more attractive.
The improvement in data collection, combined with more complexity contained within the manufacturing of contact lenses, has really revolutionized the empirical approach to fitting. The empirical approach has also surpassed the practicality of diagnostic fitting to achieve the best possible lens product for a specific eye. This empirical process increases efficiency and reduces chair time while also achieving a more ideal, patient-specific product that is capable of delivering the very best contact lens performance possible. Visual quality and quantity, patient comfort, and ocular physiology are all maximized, because the lens product ultimately produced is tailor-made to a level not possible through any other means for the unique requirements of the specific eye.
As ocular profilometry continues to improve and push the CNC lathes to their limits, and as lens design complexity continues to increase, the future of contact lens fitting will likely see the replacement of the diagnostic approach almost completely by the empirical method. CLS
REFERENCES
- van der Worp E, de Brabander J, Lubberman B, et al. Optimising RGP lens fitting in normal eyes using 3D topographic data. Cont Lens Anterior Eye. 2002. Jun;25:95-99.
- Sindt C, Bennett E, Szczotka-Flynn L, Sclafani L, Barnett M. Technical Report: Guidelines for Handling of Multipatient Contact Lenses in the Clinical Setting. Optom Vis Sci. 2020 Aug;97:544-548.
- Benoit DP, Ames KS. Diagnostic versus empirical fitting. Contact Lens Spectrum. 2010 Apr;25:12-13.
- Savini G, Schiano-Lomoriello D, Hoffer KJ. Repeatability of automatic measurements by a new anterior segment optical coherence tomographer combined with Placido topography and agreement with 2 Scheimpflug cameras. J Cataract Refract Surg. 2018 Apr;44:471-478.
- Michaud L, Barriault C, Dionne A, Karwatsky P. Empirical fitting of soft or rigid gas-permeable contact lenses for the correction of moderate to severe refractive astigmatism: a comparative study. Optometry. 2009 Jul;80:375-383.
- Maller K. Using CAD/CAM lenses for Orthokeratology. Rev Contact Lens. 2004 Apr:16. Available at https://www.yumpu.com/en/document/read/32866294/using-cad-cam-lenses-for-ortho-keratology-by-ken-maller-od . Accessed June 29, 2021.
- DeNaeyer G, Sanders DR, van der Worp E, Jedlicka J, Michaud L, Morrison S. Qualitative Assessment of Scleral Shape Patterns Using a New Wide Field Ocular Surface Elevation Topographer: The SSSG Study. J Cont Lens Res Sci. 2017 Nov;1:12-22.
- Fadel D. Scleral Lens Issues and Complications Related to a Non-optimal Fitting Relationship Between the Lens and Ocular Surface. Eye Contact Lens. 2019 May;45:152-163.
- DeNaeyer G. Scleral Shape and Its Application for Scleral Lens Fitting. Contact Lens Spectrum. 2020 Sep;35:32-34,36,37,51.