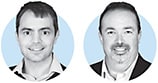
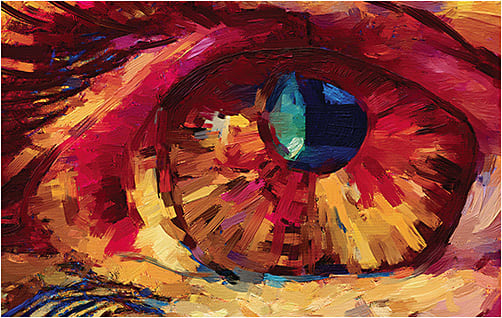
CLINICAL STUDY
Fifty-eight subjects completed a double-masked contralateral eye study. Written informed consent was obtained from all subjects, and the study protocol was approved by the Sterling Institutional Review Board in Atlanta. All subjects were habitual wearers of soft contact lenses with normal vision, normal color vision, and healthy eyes. Subjects wore the Acuvue Oasys Max 1-Day (Johnson & Johnson Vision) SW-filtering contact lens on one eye and a clear control lens on the other eye.
The SW-filtering contact lens contains a chromophore that filters up to 60% of SW light in the 380nm to 450nm portion of the visible light spectrum. Notably, it does not filter light at 460nm to 480nm, the dominant wavelengths associated with melatonin suppression and circadian rhythm entrainment.4-6
The control lens had a blue visibility tint, as most contact lenses do, and an ultraviolet light-absorbing compound but did not filter any wavelengths within the visible light spectrum. The choice of eye (right or left) for the test lens was randomized. Testing began after 25 minutes of wear to allow for adaptation.
Color appearance was quantified using chromaticity coordinates measured with a spectral radiometer within a custom-built tricolorimeter. Additive tricolorimetry, in which three primary colors (red, green, and blue [RGB]) were mixed or “balanced” using filters in the device to achieve neutral white, was used. The three primary colors selected approximate the relative balancing of red, green, and blue light that is present in computer, TV, or other digital displays.
The investigator started on the red end of the red-green axis of the tricolorimeter and confirmed that the subject monocularly perceived the color red, then gradually added green and later blue until it looked as white as possible to the subject, with no tinge of color. This process was repeated for green and blue starting points and then again for all three primaries for the fellow eye. The results were recorded as chromaticity coordinates for each eye (Table 1). Chromaticity coordinates provide a geometric representation (Figure 1) of color space that allows even small alterations in color appearance to be assessed. The results demonstrate that there is no difference between the clear and the SW-filtering lenses, out to one-thousandth of a decimal point.
n | x | y | u’ | v’ | |
SW Filtering CL | 58 | 0.345 | 0.325 | 0.222 | 0.470 |
Clear CL | 58 | 0.344 | 0.325 | 0.223 | 0.471 |
Of course, there can be significant differences between individuals in sensitivity to light and in color perception, even among people with “normal” Ishihara test results. The contralateral design also helped to ensure that interindividual differences in color perception did not influence the study. The within-subject nature of the study design served to characterize the manner in which the lens influenced the white point for each individual.
Subjects across a wide age range were tested and results between those in younger (18- to 39-year-old, n = 31) and older (40- to 65-year-old, n = 27) cohorts were compared. Older subjects might be expected to have slightly yellower crystalline lenses, which could have an additive effect on light blocking and color perception. However, there was no difference in chromaticity coordinates of subjective white point by age.
The tightly controlled and calibrated visual stimuli used in this clinical test were informative, but it is also desirable to consider other stimuli to more fully characterize the impact of the SW-filtering contact lens. In the clinical study, the three primaries were each approximately 15nm to 30nm wide—certainly not the same bandwidth as the complete visible spectrum emitted by the sun.
Even indoors, there are a variety of lighting conditions with a broader spectrum than the three primaries in the clinical study. A typical incandescent bulb, for example, would have a broader bandwidth than our stimulus. To fully understand what a contact lens filter would do to color perception, a vision model was used to evaluate the performance of the lens filter with a full spectrum of light.
MODELING COLOR PERCEPTION
For the second part of the study, five sets of hyperspectral images were used. A hyperspectral image is one in which each pixel of the image has a full spectrum of data rather than just RGB values of typical images. The image sets, most of which have previously been evaluated in the published literature, included a mix of indoor and outdoor scenes, including complex natural scenes and simpler, but color-rich images (e.g., a set of colored pencils or spools of colored thread). An outdoor scene might contain many shades of the same color and a range of colors spanning the gamut.
Before reaching the retina, light is filtered by the contact lens, the crystalline lens, and the macular pigment. The model assumed a standard observer with normal color vision, a young (20-year-old) crystalline lens of 0.38 optical density, and with average macular pigment of 0.35 optical density. The known spectrum of each image pixel was multiplied by the transmittance spectrum of the contact lens and the standard observer crystalline lens and macular pigment to estimate the image that is available to the cone photoreceptors. Data from the literature were leveraged to model the spectral responsivity of each cone class.
Humans with normal color vision have three different types of cones: L cones that are more sensitive to longer wavelengths; M cones that are more sensitive to middle wavelengths; and S cones that are more sensitive to shorter wavelengths. All of those were included in the model, based on data from the literature, and each hyperspectral image was evaluated with the observer-specific LMS values, with and without the SW filter, to generate representative cone excitation images.
Finally, the adaptation process—eyes will adapt somewhat to any filter—was also simulated by acquiring a white element in the image (clouds, or a piece of paper, for example) and re-scaling the image against this “constant white” to obtain a final, post-receptor-adapted image. Overall, there was very little change in how an image appears through a SW-filtering contact lens, as can be observed from side-by-side representational images for one set of hyperspectral images (Figure 2) and by the plotted quantitative assessments of changes in the filtered images (Figure 3).
Changes in chromatic contrast were also characterized. A pale yellow, for example, is not as far from white, in terms of chromatic contrast, as a deep purple. The change in chromatic contrast that was due to the SW filter was calculated for every pixel in every image in the dataset, generating millions of data points. From all those data points a statistical distribution of either enhancement or degradation in color was calculated. While some pixels exhibit chromatic contrast reductions, others exhibit chromatic contrast enhancement.
The predicted effects of the tested SW-filtering lenses on the gamut of colors available to a standard observer in the series of hyperspectral images indicated that the SW-filtering lenses are expected to degrade L/(L+M) chromatic contrast by 0% to 0.1% on average and to degrade S/(L+M) chromatic contrast by between 3.6% and 8.2% on average.
There are changes, so we cannot say the filter has no impact on chromatic contrast at all. Violets, blues, oranges, and yellows tend to exhibit modest degradations in chromatic contrast. However, the changes in chromatic contrast are so subtle and balanced that the qualitative impact is not easily perceived.
The published results are based on a young eye (the 20-year-old standard observer), but the sensitivity of the model to age was also tested. Changing the assumptions regarding crystalline lens age did not have much of an impact on the results. That reaffirms the findings of the clinical study, where there wasn’t much difference between the older and younger group of subjects with respect to how the contact lens filter affected color vision.
CONCLUSION
The impact of any filter on color perception depends in part on the filter, the image or stimulus, and the light source. Computer displays and other kinds of screens represent a narrower spectrum of color than occurs in the natural world, particularly outdoors in direct sunlight, which is why both clinical and modeling tests were conducted to evaluate the SW-filtering contact lenses. What we found in both studies is that, while there are very subtle changes in chromatic contrast, the effect on color perception is negligible.
This evaluation was specific to one particular SW-filtering contact lens. Every light-filtering product, whether spectacle lens, IOL, or contact lens, has its own unique transmission spectrum: Not every “blue blocker” is the same. Similarly, rigorous testing would be needed to understand the impact of other lenses on color vision. In conclusion, a subtle contact lens chromophore designed to filter SW blue-violet light did not meaningfully change color appearance for subjects who have normal color vision. CLS
References
- Lawrenson JG, Hull CC, Downie LE. The effect of blue-light blocking spectacle lenses on visual performance, macular health and the sleep-wake cycle: a systematic review of the literature. Ophthalmic Physiol Opt. 2017 Nov;37:644-654.
- Henderson BA, Grimes KJ. Blue-blocking IOLs: a complete review of the literature. Surv Ophthalmol. 2010 May-Jun;55:284-289.
- Hammond BR, Buch J, Renzi-Hammond LM, Bosten JM, Nankivil D. The effect of a short-wave filtering contact lens on color appearance. J Vis. 2023 Jan 3;23:1-13.
- Brainard GC, Hanifin JP, Rollag MD, et al. Human melatonin regulation is not mediated by the three cone photopic visual system. J Clin Endocrinol Metab. 2001 Jan;86(1):433-6.
- Brainard GC, Lewy AJ, Menaker M, et al. Effect of light wavelength on the suppression of nocturnal plasma melatonin in normal volunteers. Ann NY AcadSci. 1985 Sept;453:376-378.
- Tosini G, Ferguson I, Tsubota K. Effects of blue light on the circadian system and eye physiology. Molecular vision. 2016;22:61.