
MYOPIA IS QUICKLY becoming a serious public health issue.1 Although many questions about this phenomenon remain unanswered, increasing evidence is becoming available to help address these issues.
Myopia is thought to be driven by either axial length increase or a change in the refractive components of the eye, chiefly the cornea and crystalline lens. Eye elongation is subject to local mechanisms,2 driven by the quality of the optical image on the central retina,3 and, more importantly, the peripheral retina,4 at no more than 10º to 20º from the fovea.5
The quality of the optical image is complex and influenced by in-focus and defocused stimuli, chromatic aberration, contrast adaptation, and the spatial frequency content.6 The peripheral retinal response will generate release of neuromodulators, leading to choroidal thickness changes and scleral remodeling.7 More specifically, the visual signal modulates the rate of retinal proteoglycan synthesis with an associated impact on scleral structural integrity.8 Consequently, the biomechanical properties of the eye are modified. Softer tissue tends to elongate more than stiffer tissue. Intraocular pressure (IOP) and the convergent tensile forces exerted by the muscles during accommodation and convergence8,9 may contribute to axial length progression. It is important to note that IOP increases significantly (12 to 17 mmHg) in patients between 6 and 9 years old,10 while retrobulbar cerebrospinal pressure remains stable during this period.11
The difference between these elements is known as the translaminar pressure difference (TLPD). Lowering TLPD may influence axial elongation of the eye, especially in these younger patients who have a thinner and softer sclera.12 This may explain, in part, why myopia progression is faster and more critical before the age of 12 years.
Different approaches to slow down such progression have been studied. All methods share the same approach of influencing the retinal response and thus remodeling the sclera. The majority of approaches do this by influencing the quality of the image on the retina.
A very important element must be considered: This retinal response is individual, each person being endowed with a threshold at which their retina reacts.13 The intensity of the signal, defined by the amount of defocus generated and the area of impact on the retina, must therefore be modulated according to the individual. A second element must also be taken into account: In animal models14 as well as in humans,15 there is a dose-response mechanism (i.e., the greater the intensity of the optical signal, the greater the retinal response).
Thus, the optical devices designed for myopia management must be customized to the individual and must generate the highest level of defocus, especially for higher myopes and those progressing at a faster rate.
ORTHOKERATOLOGY: APPLICATION FOR MYOPIA MANAGEMENT
Orthokeratology (ortho-k) was not developed to control the axial elongation of the eye but as a substitute for optical devices to reduce myopia on a temporary basis.16 To achieve this goal, ortho-k lenses must reshape the eye surface profile by using the hydraulic force of tears to flatten the central cornea.17
In order to minimize the complications encountered in the past (erosions, permanent corneal warpage, microbial keratitis),18 the modern ortho-k lens architecture is based on a reverse-geometry design.19 This implies that the central lens curvature, which is flatter than the cornea, must be balanced by a steeper one, called a reservoir, and a series of alignment curves, allowing a smooth landing on the cornea and essential tear exchange.
The corneal profile modification is mostly attributed to the compression or the distention of the epithelial cells. The other layers of the cornea being minimally affected, with the exception of an increased number of active keratocytes and a reduction in nerve density of the subbasal plexus centrally.20 There is no exchange of fluids between the cells or migration of the latter.
When the positive (flattening) and negative (under the reservoir) pressure is released, the epithelial cells tend to return to their original shape. The ortho-k effect can only be temporary, although the maximum effect is obtained after seven to 10 days of wear, whereas the return to the initial conditions can take seven days, but may last for several weeks, especially when higher myopia has been corrected.21
DUAL-FOCUS OPTICS
This optical effect of ortho-k can be described as dual focus. The optical stimulus generated by the central flattened zone should be focused on the retina to ensure clear vision, without residual refractive error, as long as one remains within the U.S. Food and Drug Administration (FDA) approval limits up to –6D.22 The optical image generated by the steeper peripheral cornea is in myopic defocus, accompanied by a significant increase in higher-order aberrations (HOAs), notably positive spherical aberration and coma. The latter is considered an important factor in ortho-k to manage eye elongation effectively.23
The retina can interpret contradictory optical signals simultaneously.9 Response to these signals will dictate whether the eye resists the elongation or if, on the contrary, it is stimulated to grow. The ultimate outcome relates to a feedback loop using visual cues as an error signal to regulate eye growth.
MYOPIA CORRECTION TO AXIAL LENGTH MANAGEMENT
Considering that ortho-k generates both clear (in focus) and blurred (defocus) images, maximizing the former and minimizing the latter ensures functional and comfortable vision for the patient. For this reason, most ortho-k lenses favor a design with a wide central treatment zone (6mm) to push the more convex areas peripherally beyond the pupil to minimize their presence (Figure 1). These more convex areas are associated with the perception of halos and can contribute to glare, depending on the ambient lighting.
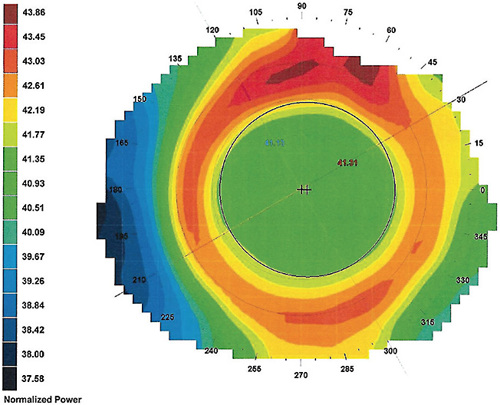
The treatment zone diameter is inversely related to the target refractive change, based on the Munnerlyn formula,21 considering a correction up to –4D and three months of lens wear. In recent months, there is evidence that the use of smaller treatment zones is associated with better results in myopia control,24 particularly when the areas generating defocus and HOAs are clearly located within the pupillary area, thus optimizing the peripheral retina exposure to favorable optical signals (Figure 2).25
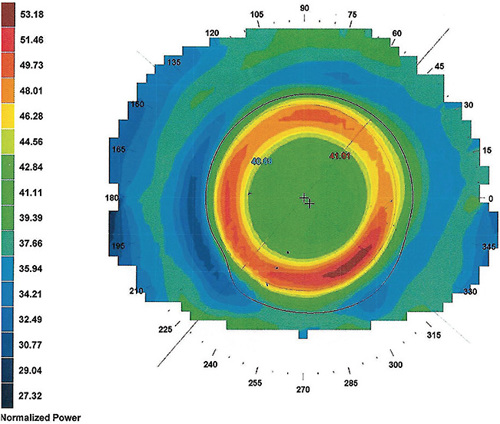
It is important to note that the results vary between individuals. The same lens applied to two corneas that have similar central curvatures will not give the same result, due to variations in their toricity, elevation, eccentricity, etc. It is important to evaluate the impact of the lens on the eye by analyzing the tangential maps before and after the ortho-k lens fitting in order to validate the position of each zone, its dimensions, and any possible decentration.26
Slight decentration may be acceptable, as it may be associated with a better performance in terms of myopic management27 (Figure 3). Indeed, the fact that the lens is slightly decentered downward implies a greater dose of optical aberrations entering superiorly, where the entrance pupil is located, therefore improving overall control.28
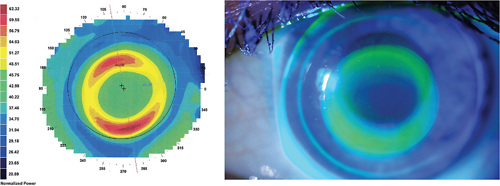
DOSE MATTERS
The control of the dose of optical aberrations is certainly the most important factor arguing for a full customization of lens designs used in ortho-k.
It is generally agreed that patients who have low myopia are associated with reduced effectiveness of ortho-k on axial length progression. This is because the power generated under the reservoir is usually equal to the myopic correction made, considering a standard ortho-k lens design (1:1 ratio). This means that a –1.50D correction will generate +1.50D under the reservoir.
This level of addition is less than what is normally desirable and may be ineffective for myopia management, especially since a small myopic correction in ortho-k is usually associated with a larger treatment zone area. Thus, the region of increased positive power is pushed to the margin or outside the pupil, which also contributes to reduce the efficiency of the treatment.
Some standard ortho-k lens designs already offer smaller treatment zone areas, so they would be favored when used for myopia management. The best option, however, is to use software that can establish each of the lens parameters to be prescribed, optimizing their corneal and optical impacts. Under such circumstances, it is possible to generate an add of +6D to +8D while correcting only –1.50D.
Mastering lens design software may take a few days, weeks, or months. It does not entirely depend on the practitioner’s facility with computers and software, but first and foremost on the knowledge of the prescriber about ortho-k and lens dynamics on the eye. Most software suppliers offer training on how to use their products and how to approach lens customization with success.
LENS DIFFERENCES
In theory as in practice, ortho-k lenses designed for myopia management are different from those used for the simple correction of this refractive error. In simple terms, the goal is to adequately correct distance vision while generating a maximum amount of defocus, HOAs, and other elements that slow down myopia progression and axial length elongation, primarily by manipulating the number of curves, the depth of the reservoir, and the ratio between the treatment zone and the pupillary area.
The lens should be larger and offer less tear exchange to optimize the effect of hydraulic pressure under the lens. To better follow the contour of the cornea, additional curves are usually required. Table 1 summarizes the major differences between custom and conventional lenses (manufacturer’s ortho-k designs).
Differences Between Lens Designs | ||
MYOPIA MANAGEMENT | MYOPIA CORRECTION | |
Jessen Factor | Habitually higher (superior to 1.00 and inferior to 3.00) | Lower (1.00 or less); 0.75 in general |
# Curves | Five or more | Four to five in general |
Diameter | Larger diameter. Must cover 90% to 95% of the visible cornea (HVID). | 80% to 90% of the visible cornea. |
Central zone diameter | Smaller (5.0mm-6.00mm in general)Proportional to the pupil area Aim to enhance peripheral defocus and positive spherical aberration. |
Larger (6.00mm in general) Aim to alleviate haloes. |
Back surface positive asphericity | Higher (> 1.0) to generate a deeper reservoir. Jessen Factor must be modified accordingly (higher as well). | Rarely used |
Reservoir (reserve curve) | Customizable to enhance corneal molding, especially in low myopes (going over 1:1 ratio) | Fixed versus refractive error (1:1 ratio—myopia corrected versus +power-generated) |
Landing zone | Toric/quadrant-specific to enhance centrationSteeper than K | Toric to enhance centration On K, or flatter than K |
Peripheral curves | Steeper to enhance seal-off effect | Flatter to allow tear exchange |
Overall lens sag | Higher | Lower |
Lens movement (slip lamp) | Reduced, fluorescein exchange almost stagnant | Regular—fluorescein exchange as habitually seen with RC lenses |
Recreated with permission from The Dougmar Publishing Group. |
Customize Your Approach The ortho-k lens-fitting methods that were developed for myopic correction should be reviewed when dealing with myopia or axial length management. Children are now fitted more often than adults, for whom traditional ortho-k designs were developed. It is necessary to optimize the dose of positive peripheral power delivered to the eye by modifying the optical image on the retina, which requires a custom design approach.
To this end, lenses must be modulated according to not only individual physiological criteria, but also patient behavior. An 8-year-old moderately myopic child who has rapid progression and small pupils should not be fitted with the same lens, or with the same parameters, as his or her 17-year-old brother, who has larger pupils and whose high myopia has stabilized but who also drives at night.
In the latter case, a treatment zone that is too small could generate annoying haloes, which is less well tolerated than it is at a young age. Full customization is needed for the younger patient to maintain good management of axial length elongation without compromising distance vision or visual comfort.
Ideal Candidates If every myopic child deserves to be provided with custom lenses, it remains that the patients described in Table 2 are those who will benefit the most.
CLINICAL FEATURE | JUSTIFICATION |
---|---|
Low myopic (< 2.00D) | Need to go over 1:1 ratio |
Central corneal astigmatism > 2D | May need toric base curve |
Corneal elevation difference at 7mm (> 20μm) | Need toric/quadrant-specific peripheries |
Treatment zone versus pupil area | To optimize the ratio between these two elements (customized for each patient, not fixed as large or small diameter) |
Number of lens curves | Increasing from four to six or seven curves may optimize lens-to-cornea relationship (overall outcome, centration, etc.). |
Lens diameter | Must cover 95% of the visible iris diameter. Better if customized to the patient’s parameter. |
Lens sagittal depth | Modifiable with customization to help troubleshooting (smiley face, frowny face, central island, etc.) |
CONCLUSION
The benefits of a custom approach to ortho-k lens design have been demonstrated. Each myopic child is unique, and his or her risk of developing eye disease in the future depends on several factors, most notably the ultimate level of myopia. It is therefore preferable to adapt the lens to the patient rather than the other way around.
This logic should also apply to the global approach to myopic management. Each case must be carefully analyzed, and strategies must be proposed that correspond to the patient’s condition, lifestyle, available budget, etc. For this reason, it is important that myopia management using ortho-k should, like other methods, be addressed one child at a time. CLS
References
- Resnikoff S, Jonas JB, Friedman D, et al. Myopia - A 21st Century Public Health Issue. Invest Ophthalmol Vis Sci. 2019 Feb 28;60:Mi-Mii.
- Smith EL 3rd, Hung LF, Huang J, Blasdel TL, Humbird TL, Bockhorst KH. Effects of optical defocus on refractive development in monkeys: evidence for local, regionally selective mechanisms. Invest Ophthalmol Vis Sci. 2010 Aug;51:3864-3873.
- Chung K, Mohidin N, O’Leary DJ. Undercorrection of myopia enhances rather than inhibits myopia progression. Vision Res. 2002 Oct;42:2555-2559.
- Benavente- Pérez A, Nour A, Troilo D. Axial eye growth and refractive error development can be modified by exposing the peripheral retina to relative myopic or hyperopic defocus. Invest Ophthalmol Vis Sci. 2014 Sep 4;55:6765-6773.
- Maiello G, Walker L, Bex PJ, Vera-Diaz FA. Blur perception throughout the visual field in myopia and emmetropia. J Vis. 2017 May1;17:3.
- Hung GK, Ciuffreda KJ. Incremental retinal-defocus theory of myopia development--schematic analysis and computer simulation. Comput Biol Med. 2007 Jul;37:930-946.
- Boote C, Sigal IA, Grytz R, Hua Y, Nguyen TD, Girard MA. Scleral structure and biomechanics. Prog Retin Eye Res. 2020 Jan;74:100773.
- Holladay JT. Method of mitigating myopia development and related instrumentation. US Patent #US11298263B2. 2022 Apr 12. Available at patents.google.com/patent/US11298263B2/en?q=METHOD+OF+MITIGATING+MYOPIA+DEVELOPMENT+AND+RELATED+INSTRUMENTATION&oq=METHOD+OF+MITIGATING+MYOPIA+DEVELOPMENT+AND+RELATED+INSTRUMENTATION . Accessed Jan. 30, 2023.
- Troilo D, Smith EL 3rd, Nickla DL, et al. IMI - Report on Experimental Models of Emmetropization and Myopia. Invest Ophthalmol Vis Sci. 2019 Feb 28;60:M31-M88.
- Dusek WA, Pierscionek BK, McClelland JF. Age variations in intraocular pressure in a cohort of healthy Austrian school children. Eye (Lond). 2012 Jun;26:841-845.
- Avery RA, Shah SS, Licht DJ, et al. Reference range for cerebrospinal fluid opening pressure in children. N Engl J Med. 2010 Aug 26;363:891-893.
- Sunita M, Manisha S, Sanjeev M, Ravi KS, Aarzoo J, Ajai A. Anatomical and Clinical Characteristics of Paediatric and Adult Eyes. Nat J Clin Anat. 2021 Jan 27;10:5-9.
- Tepelus TC, Schaeffel F. Individual set-point and gain of emmetropization in chickens. Vision Res. 2010 Jan;50:57-64.
- Tse DY, To CH. Graded competing regional myopic and hyperopic defocus produce summated emmetropization set points in chick. Invest Ophthalmol Vis Sci. 2011 Oct 17;52:8056-8062.
- Walline JJ, Walker MK, Mutti DO, et al. Effect of High Add Power, Medium Add Power, or Single-Vision Contact Lenses on Myopia Progression in Children: The BLINK Randomized Clinical Trial. JAMA. 2020 Aug 11;324:571-580.
- Bullimore MA, Johnson LA. Overnight orthokeratology. Cont Lens Anterior Eye. 2020 Aug;43:322-332.
- Mountford, J. A model of forces acting in orthokeratology. In Orthokeratology, principles and practice. London: Butterworth-Heinemann; 2004: 269-299.
- Swarbrick HA. Orthokeratology review and update. Clin Exp Optom. 2006 May;89:124-143.
- Mountford J. Design variables and fitting philosophies of reverse geometry lenses. In: Orthokeratology, principles and practice. London: Butterworth-Heinemann; 2004:69-109.
- Sánchez-García A, Ariza MA, Büchler P, Molina-Martin A, Piñero DP. Structural changes associated to orthokeratology: A systematic review. Cont Lens Anterior Eye. 2021 Aug;44:101371.
- Vincent SJ, Cho P, Chan KY, et al. CLEAR - Orthokeratology. Cont Lens Anterior Eye. 2021 Apr;44:240-269.
- Potter RT. Outside-the-box Orthokeratology. Contact Lens Spectrum. 2018 Dec;33:28-31. Available at clspectrum.com/issues/2018/december-2018/outside-the-box-orthokeratology . Accessed Jan. 30, 2023.
- Hiraoka T, Kakita T, Okamoto F, Oshika T. Influence of ocular wavefront aberrations on axial length elongation in myopic children treated with overnight orthokeratology. Ophthalmology. 2015 Jan;122:93-100.
- Michaud L, Simard P, Marcotte-Collard R, et al. The Montreal Experience: A Retrospective Study Part I—Basic Principles and Treatment Algorithm. Appl Sci. 2021 Aug 13;11:7455.
- Pauné J, Fonts S, Rodríguez L, Queirós A. The Role of Back Optic Zone Diameter in Myopia Control with Orthokeratology Lenses. J Clin Med. 2021 Jan 18;10:336.
- Marcotte-Collard R, Simard P, Michaud L. Analysis of Two Orthokeratology Lens Designs and Comparison of Their Optical Effects on the Cornea. Eye Contact Lens. 2018 Sep;44:322-329.
- Lin W, Li N, Gu T, et al. The treatment zone size and its decentration influence axial elongation in children with orthokeratology treatment. BMC Ophthalmol. 2021 Oct;21:362.
- Chen R, Chen Y, Lipson M, et al. The Effect of Treatment Zone Decentration on Myopic Progression during Orthokeratology. Curr Eye Res. 2020 May;45:645-651.
- Michaud L, Simard P, Marcotte-Collard R, Ouzzani M. Managing Myopia: One Child at a Time. Toronto: Dougmar Publishers; 2022.