
Final in a series of four CE activities for 2023.
LEARNING METHOD AND MEDIUM
This educational activity consists of a written article and 20 study questions. The participant should, in order, read the Activity Description listed at the beginning of this activity, read the material, answer all questions in the post test, and then complete the Activity Evaluation/Credit Request form. To receive credit for this activity, please follow the instructions provided below in the section titled To Obtain CE Credit. This educational activity should take a maximum of 2 hours to complete.
CONTENT SOURCE
This continuing education (CE) activity captures key statistics and insights from contributing faculty.
ACTIVITY DESCRIPTION
The goal of this article is to show that technology is forecast to fulfill the need for automated lens parameter selection for specialty and smart contact lenses having physical properties and that it may be less forgiving than modern single use contact lenses.
TARGET AUDIENCE
This educational activity is intended for optometrists, contact lens specialists, and other eyecare professionals.
ACCREDITATION DESIGNATION STATEMENT
This course is COPE approved for 2 hours of CE credit.COPE Course ID: 87485-CL
DISCLOSURES
Jerome A. Legerton, OD, MS, MBA, reports no conflicts of interest.
DISCLOSURE ATTESTATION
The contributing faculty member has attested to the following:
- That the relationships/affiliations noted will not bias or otherwise influence his involvement in this activity;
- That practice recommendations given relevant to the companies with whom he has relationships/affiliations will be supported by the best available evidence or, absent evidence, will be consistent with generally accepted medical practice;
- That all reasonable clinical alternatives will be discussed when making practice recommendations.
TO OBTAIN CE CREDIT
To obtain COPE CE credit and instant certificate processing for this activity, read the material in its entirety and consult referenced sources as necessary. Please take the post test and evaluation by following the link below and clicking on the CE Information tab.
Upon passing the test, you will immediately receive a printable PDF version of your course certificate for COPE credit. On the last day of the month, all course results will be forwarded to ARBO with your OE tracker number, and your records will be updated. You must score 70% or higher to receive credit for this activity. Please make sure that you take the online post test and evaluation on a device that has printing capabilities.
NO-FEE CONTINUING EDUCATION
There are no fees for participating in and receiving credit for this CE activity.
DISCLAIMER
The views and opinions expressed in this educational activity are those of the faculty and do not necessarily represent the views of Contact Lens Spectrum. This activity is copyrighted to Broadcastmed LLC ©2023. All rights reserved.
This activity is supported by unrestricted educational grants from Art Optical and Contamac.
CE Questions? Contact CE@pentavisionmedia.com for help.
RELEASE DATE: NOVEMBER 1, 2023
EXPIRATION DATE: OCTOBER 4, 2026
SMART CONTACT LENSES and eyewear have been the topic of discussion for a decade, but still remain beyond our clinical reach. At the same time, refractive error regulation through corneal reshaping, peripheral defocus lenses, and pharmaceutical intervention have become the megatrend that was forecast two decades ago.
Devices and contact lenses for irregular corneal astigmatism are now an established modality, with the forecast of an increased use of custom soft lenses as an alternative to scleral or hybrid lenses. Contact lenses for presbyopia are becoming more accepted and delivering useful vision at distance and near. Technology-driven prescribing is a common component to potentially higher success and higher efficacy in all four categories.
One model for being a visionary or futurist involves selecting trendlines and observing where they intersect. The trends of managed care, higher patient volumes per day, cost containment requirements driven by inflationary pressure, consumers having reduced disposable income for out-of-pocket expenses, a reduction in the number of independent practices, higher salaries for employed eyecare practitioners (ECPs), and a general shortage of physicians relative to the number of ophthalmic clinics, coupled with the desired expansion by corporate ophthalmic delivery companies, all point to the need to deliver contact lens care more efficiently and accurately.
The trends on the product side include excellent micron scale diamond turning and nanoscale molding technology for contact lenses, ultra-high Dk contact lens materials, advancements in material science for surface modification, and quantum leaps in lens metrology technology that feed back to the precision of manufacturing contact lenses. Add to this the trends in instrument technology for measuring ocular contour and the lower and higher-order aberrations (HOAs) of the eye, all of which point to a future defined by the phrase “technology-driven contact lens prescribing.”
Let’s take a closer look at these trends in the context of what is available today and what might be available in the near future.
TODAY AND MAYBE TOMORROW
The recent strategic shift by MojoVision to halt its MojoLens development and focus on miniaturization of electronic components—after investing more than $200 million—was not the first retreat from the challenges of smart contact lenses. Alcon and Alphabet also invested millions to achieve health- and wellness-sensing lenses. The same is true for Johnson and Johnson Vision Care, which partnered with IBM to develop accommodative contact lenses for presbyopia.
The pursuit of active and passive drug delivery contact lenses has received a significant cumulative investment by several companies to meet a clearly understood medical need. The first product in this category has been launched and the development of other drug delivery products is showing up in the news. There are multiple strategies for drug delivery, including the simple placement of the pharmaceutical and potentially a reformulated version within the bowl of a scleral lens or using a novel lens material that will not uptake the pharmaceutical molecules.
One company has put more than a decade of development into a passive smart lens, an optical solution to assist in reducing the size and weight of extended reality display eyewear. This remains some distance from the finish line. Clinical testing is being done on another lens that shows the promise of eye-borne optics for normally sighted and visually impaired users.1,2
All of these efforts have delivered valuable pieces of the smart lens puzzle, yet there are no products available that fulfill the promises of the original missions of these companies. Some of the companies appear to have halted their efforts altogether, and others continue on and are joined by new companies. What are the apparent obstacles?
Most practitioners will agree that comfort is king when it comes to contact lenses. Contact lenses, like all products, must be usable as well as useful. Comfort is a primary ingredient in usability. Vision, health, and convenience are the other three legs on the stool of usability.
Most smart lenses are, by necessity, thicker or may have a higher modulus and stiffness. As such, they are not as forgiving in circumferential draping as one-size-fits-all disposable lenses. The ability to achieve all-day comfortable wear is more challenging with stiffer lenses.
The selection of parameters is, for the same reason, also more challenging, and the guidelines for fitting look like those from the early 1980s due to the need for more permutations in diameter, base curve radius, and sagittal depth to overcome the absence of forgiveness in thin low-modulus lenses.
This challenge is exacerbated if the form factor is a scleral lens and, even worse, if it is a hybrid-like lens or a scleral lens that is substantially thicker. Gravity alone causes a greater failure of the lens to center over the visual axis, and increased impingement over a section of the sclera is particularly challenging.
The primary take-away is that smart contact lenses demand technology-driven prescribing as soon as they depart from one-size-fits-all, particularly if they are intended for mass market uptake. The prescribing of a more challenging lens must not become the bottleneck hindering commercial success for a smart lens company. Hence, technology-driven prescribing is a critical success factor.
IN THE ERA OF DIGITAL HEALTH AND TELEMEDICINE
Art generally precedes scientific understanding. Practitioners prescribing contact lenses 50 years ago were required to apply a good portion of art to their scientific understanding of corneal shape, lid interaction, tear exchange, and optical correction needs when selecting contact lens parameters. Over time, clinical instrument metrology improved and ECPs were able to add the Placido disk keratoscope to our keratometry. Then, we enjoyed the availability of corneal topographers and their software for presenting power and elevation maps.
Now, eyecare practitioners have tomography instrumentation to help them understand ocular contour to assist in designing the landing zones of scleral lenses and determine the sagittal depth of the eye at a key chord diameter, allowing them to empirically select the sagittal depth of scleral lenses to achieve pre-compression apical clearance.
Some opinion leaders in the field of corneal refractive therapy/orthokeratology believe that the majority of lenses can be empirically calculated by software using corneal topography, horizontal visible iris diameter (HVID), confirmed flat keratometry from auto keratometry, and the manifest refraction. In other words, clinical data may be used to suggest an initial lens. A remote observation of the fluorescein pattern should be followed by a post 30-minute closed eye wearing period.
Then, lens removal topography may be used to determine the accuracy of the empirically suggested parameters and the resultant closed-eye centration of the lens. All of this can be accomplished without being present with the patient.
Remote parameter selection and prescribing of orthokeratology lenses is further made possible by a remotely operated digital slit lamp. Figure 1 is an image of a commercially available slit lamp that is intended for remote operation.
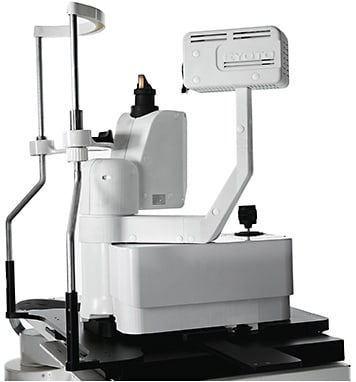
Digital health and telemedicine instrument technology is partially driven by the needs of corporate vision care in the United Kingdom and Europe. Some corporate providers have scores of what they call “dark stores” due to the shortage of optometrists. Similar groups in the U.S. are experiencing an inability to staff all of their locations and expand their number consistent with their strategic plans because of a lack of practitioners who are available, affordable, or both.
At the same time, several companies are developing next-generation instrument suites and software with the objective of integrating clinical metrology with databases and digital case history input to support the decision-making of remote practitioners. These remote practitioners may be simultaneously managing active patients in multiple locations with the support of ancillary personnel.
It is forecast that the parameters of an orthokeratology contact lens, a specialty soft lens including free-form surfaces and wavefront optics, a hybrid lens, a scleral lens, or a less forgiving smart lens may be prescribed by information obtained by clinical instrumentation and observed by remotely operated clinical instruments, including biomicroscopes. This will be a significant achievement in the application of high tech in the world of digital health and telemedicine.
CLINICAL INSTRUMENTATION AND THE LINE OF TENSION
The starting point in understanding the value of instrumentation is determining what clinical metrics drive the parameter selection decisions. Lens manufacturers assist in this area by creating prescribing systems that teach the metrics that drive determination of each parameter and the relationship between the measured element and said parameter.
Table 1 shows examples of clinical measurements and corresponding lens parameters for an orthokeratology lens. Table 2 reports examples of clinical measurements and corresponding parameters for a scleral lens.
CLINICAL METRIC | PREDICTED CONTACT LENS PARAMETER | INSTRUMENT TECHNOLOGY | AVAILABILITY OF INSTRUMENTATION |
Apical corneal radius | Base curve radius | Auto keratometry | Broad |
Corneal diameter | Overall lens diameter | Corneal topography | Broad |
Corneal elevation data | Radii and widths of reverse curve and alignment curve; Return zone depths and landing zone angles | Corneal topography | Broad |
Manifest refraction | Base curve radius | Refraction suite | Broad |
CLINICAL METRIC | PREDICTED CONTACT LENS PARAMETER | INSTRUMENT TECHNOLOGY | AVAILABILITY OF INSTRUMENTATION |
Apical corneal radius | Base curve radius | Auto keratometry | Broad |
Corneal diameter | Overall lens diameter | Corneal topography | Broad |
Corneal elevation data | Peripheral corneal zone sagittal depth | Corneal topography | Broad |
Manifest refraction | Base curve radius | Refraction suite | Broad |
Over-refraction | Lens power | Refraction suite | Broad |
Aberrometry and simultaneous registration capture | Higher-order aberration correction | Aberrometry coupled with simultaneous registration capture | Commercially available and limited |
Orientation mark position for low-order cylinder axis | Cylinder axis when manufactured | Biomicroscopy | Broad for non-remote prescribing; Limited for remote |
Tomography | Sagittal depth of scleral lens; Meridional sagittal depth in limbal clearance zone and landing zones | Tomography including fringe topography, optical coherence tomography, or Scheimpflug technology | Commercially available and limited |
The clear gap is in the number of available instruments for fully automated measurement and communication of the needed information for parameter selection algorithms for lenses that extend beyond the limbus. Several companies are developing machine-learning systems for evaluating clinical data and communicating these data, analysis results, and suggested diagnosis and treatment alternatives to practitioners.
A major problem for contact lens manufacturers is the small market penetration of instruments required for technology-driven parameter selection for lenses that extend beyond the limbus. For example, after more than a decade of strong market growth of scleral lenses, it is estimated that there are fewer than 200 instruments in practitioners’ offices for measuring full circumferential scleral contour. Some of these instruments are challenging to use for image capture, others require taking multiple images that require stitching by the software, while others carry a cost that exceeds the emotional decision-making level of the average practitioner or the corporate entity that needs to purchase multiple units.
This is definitely a case of good being the enemy of best. Most practitioners believe their skill and ability to prescribe lenses without additional instrumentation is good enough. The common policy of “guaranteed fit” that allows for multiple lens reorders also drives the avoidance of purchasing instrumentation that may be useful in achieving a higher percentage of initial success. First lens success reduces practitioner time and cost, laboratory cost, patient time, and the cost of additional visits to the practitioner.
Good as an enemy of best in the case of the contact lens industry may also be phrased as “good enough is the enemy of sound economics.” Most industries work to lower the costs of their goods and services over time by anticipating that a reduced cost to the consumer will increase the utilization of services and, in the end, provide greater economic value to both providers and consumers. This objective becomes an imperative if, at the same time, there is a belief that an unmet need exists or that the market is underserved.
Corporate vision care delivery systems are perceived as the aggregate providers of care and are the primary focus of digital health and telemedicine enablers. It is possible that the corporate vision care delivery systems will be the early adopters and innovators of technology-driven prescribing of specialty lenses, including orthokeratology, specialty soft, scleral, and smart contact lenses. The tipping point may depend on the results of comparing first lens success, total clinical time, and patient satisfaction using remote data access for parameter selection and follow-up assessments versus traditional lower-technology/higher-art parameter selection and case management.
Instruments are available today for measuring all of the clinical metrics for orthokeratology contact lenses, and the number of practitioners who own the instruments is at a critical mass for empirical parameter selection. This is not the case for measurements of scleral contour and sagittal depth of the eye outside the limbus for scleral, hybrid, custom soft, and stiffer or more complex smart lens parameter selection.
Astute lens designers use topography differently than practitioners. Historically, practitioners were first trained to use power maps for fitting shape while designers used elevation maps to determine the sagittal depth of lenses. There is a great need for practitioners to transition to looking at elevation maps to understand where the cornea is actually deeper and where it is shallower.
The transition to seeing the cornea three dimensionally in terms of elevation, versus looking at power values or colors, paves the way for understanding the importance of rotationally asymmetric corneal lenses for improving lens centration and treatment. Figure 2 is a power map of a cornea; Figure 3 is an elevation map of the same cornea.
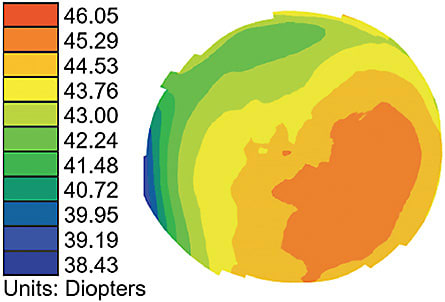
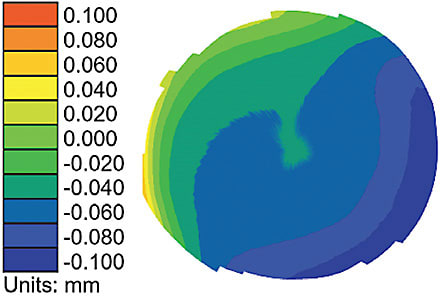
When a practitioner is only presented with the power map and asked to identify the highest area of the cornea, they most often point to the highest power. And yet, the elevation map shows that the deepest area of the cornea corresponds to the highest power.
It is established that the cornea is asymmetric and that lenses decenter in the direction of the deepest corneal area if the lenses are not designed to be asymmetric. Next-generation orthokeratology lenses have been designed and developed that employ rotationally asymmetric geometry to assist in centration and, thus, enhanced treatment.3
WHAT ABOUT LENS MATERIALS AND METHODS OF MANUFACTURING?
Currently, custom soft lenses are manufactured using diamond-turning lathes with hydrogel or silicone hydrogel buttons that have oxygen permeability of less than 35 Dk and less than 80 Dk, respectively. The thicker lens profiles result in an oxygen transmissibility (Dk/t) that is lower than the Holden-Mertz criteria for open eye wearing.4
The high manufacturing cost per lens, combined with the low oxygen transmissibility, has limited the prescription rate for this category of specialty lenses. At the same time, the standard of care for corneal cross-linking for adolescent and young adult patients with a first diagnosis of keratoconus appears to be reducing the prevalence of severe irregular corneal astigmatism. This factor, along with the relatively higher patient acceptance for a soft lens modality over a scleral lens modality, supports the greater future need for custom soft lenses.5
Two material strategies hold promise for custom soft lenses for irregular corneal astigmatism. The first is a higher-Dk silicone hydrogel that is moldable using custom molds. A material having a Dk of 130 or higher and a lens thickness of 0.50mm will then have a Dk/t of 26 and will approach the transmissibility of the Holden-Mertz criteria for open eye wearing.
The custom molds may also carry a surface profile to enable HOA correction—in addition to low-order cylindrical correction—along with required lens stabilization features. These lenses may be successful in normal thicknesses as the HOA correction becomes more refined.
The second strategy is to use an ultra-high Dk material like lemafocon A. This silicone elastomer material is fully molded to a nano-scale accuracy and has a Dk of 778. It was first selected and developed for a lens in which a two-state light polarizing filter is placed inside the lens in a patented multilayer molding process.5
The same material and lens stabilization designs hold promise for lenses for irregular corneal astigmatism with or without the addition of HOA correction. A thin lens design similar to conventional custom soft lenses for irregular astigmatism is in development.
Instrumentation is available for simultaneously measuring lens registration relative to pupil center and residual lens-eye HOAs.6 Predicate rotationally stabilized and design-defined lenses have registration marks, as illustrated in Figure 4. The measured HOAs and registration measurements are then converted to a point file for diamond turning a rigid or soft lens button or mold to produce a wavefront-guided contact lens.
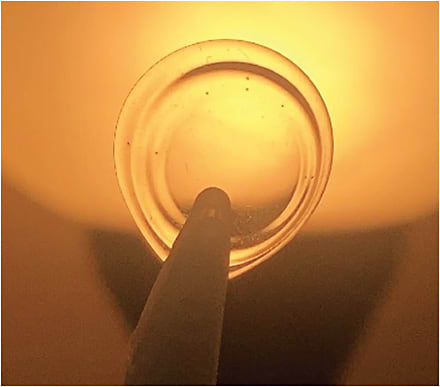
There is commercially available technology for simultaneous HOAs and registration measurement for the conversion to cutting files for wavefront-guided contact lenses. HOA-correcting lenses may also be molded using custom molds having a surface that contains the HOA features.
There are two challenges for hydrogel and silicone hydrogel materials for wavefront-guided contact lenses. The first is the radial and linear expansion factors along with their variances. HOA features are most often sub-micron, and the variance in the expansion factors has potential to cause the features to vary beyond their useful level for vision improvement. Expansion-free hydrogel and silicone hydrogel materials are more useful for wavefront-guided contact lenses due to the lower variance and greater likelihood of producing the intended HOA correction.
The same is true for the second challenge, environmentally induced dimensional instability, that is generally greater in lenses having higher water content. The dimensional changes may be of the same order of magnitude as the HOA features intended to improve the retinal image. These factors suggest that the best hydrogel or silicone hydrogel material for wavefront-guided contact lenses is relatively expansion-free and lower in water content.
The lemafocon A material has less than 1% water and is expansion-free in its manufacturing. The problems of variance in radial and linear expansion and environmentally induced dimensional stability are thus intrinsically reduced.
An additional challenge in wavefront-guided contact lenses is lens deformation caused by the use of monocurve or bicurve lens designs in which the base curve is substantially longer than the apical radius of the cornea. This is standard design practice with soft contact lenses in which the average apical radius of human corneas is about 7.8mm and the average contact lens base curve is about 8.5mm.
Even with this average departure of about 3.50D, there is no measurable tear lens power with the soft lens on eye. The average soft lens is also deeper than the average eye at the sagittal depth of its overall diameter. Hence, there must be resultant positive edge strain.7 The departure of the base curve radius from that of the eye and the departure of the sagittal depth and edge strain suggests that lens deformation is the rule rather than the exception to the rule.7
Lens designs have been suggested for wavefront-guided lenses wherein the base curve radius is selected to be closer to the apical radius of the eye in an attempt to reduce deformation in the optic zone region of the lens.8
The lemafocon A lens material is currently molded using reusable diamond-turned molds. These molds incorporate an orientation stabilizing feature along with low-order aberration and HOA correction surfaces. In this manner, the output from aberrometers that simultaneously capture the lens registration marks and the lens-eye wavefront refraction may be used to calculate the point file for molding a wavefront-guided contact lens. This system is expected to produce a monthly disposable high Dk/t HOA-correcting soft lens for irregular astigmatism that may deliver the retina-brain limitation of visual acuity for those who seek to have it.
FIRST STEPS IN TECHNOLOGY-DRIVEN PRESCRIBING
van der Worp and co-workers studied available lenses to compare the sagittal depth in an effort to create a means of knowing which brands of lenses may be more suitable for eyes that are at different places on the continuum of sagittal depths.9 One shortcoming of this first exercise is the variance in overall diameter renders the use of the measured overall sagittal depths less useful. There is a need to create a common chord diameter for which the sagittal depth is known to make the matching of the known sagittal depth of an eye more appropriate for selecting a lens from a collection of lenses having a known sagittal depth.
For example, if the sagittal depths of all lenses at the common chord of 14.0mm or 13.8mm are known, they may be more easily rationalized or ordered when the sagittal depth of an eye is known for the same chord. The impact of some lenses having larger overall diameters is negligible compared to the need to know the sagittal depth of all lenses at a common chord.
The advent of the use of optical coherence tomography to measure the sagittal depth of lenses at an equivalent specific chord provides the means for creating a database of all commercially available lenses. Then an algorithm that uses the measurement of the sagittal depth of every eye at a specific chord may be useful for selecting a lens that is expected to have the best lens-to-eye relationship with regard to edge strain. An intelligent system for predicting lens parameters may also incorporate the measured apical radius of the eye, measured HVID, lens base curve radius, lens modulus, and the harmonic mean thickness of lenses.
High-tech parameter selection may provide input to practitioners that supports the best lens-to-eye relationship potential. This scientific approach may be accepted or may be lost to other forces like competitive pricing, consumer pull-through, or the use of the “Pepsi Challenge,” where different lenses are placed on the right and left eyes and patients express their preference.
The geometric diversity of human eyes is significant. Figure 5 is the graphic representation of data from 135 right eyes of primarily Caucasian subjects using an early fringe topography technology. Note that the difference in sagittal depth of this population of eyes at a chord of 14mm is more than 2.5mm. Granted, the design input for a viable product does not need to address six standard deviations. Even so, the sagittal depth range at a common chord diameter is significant. The geometric diversity of human eyes suggests that knowing the sagittal depths of all commercial lenses and measuring a prospective eye and selecting a lens from a database of known sagittal depths is a first step in technology-driven prescribing.
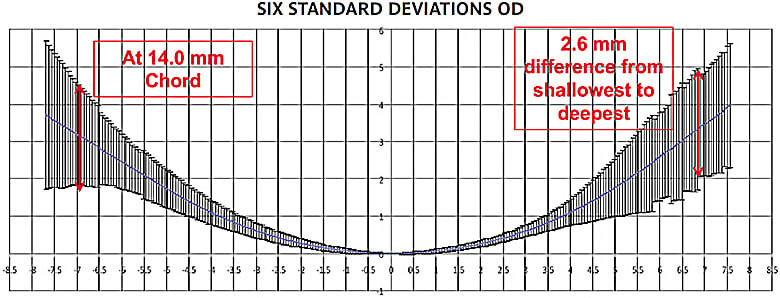
Yu and colleagues studied a smaller population of eyes using a profilometer with a corneal/scleral profiling software to study the circumferential elevation, local slope, or curvature differences of the sclera at a chord of 16mm.10 They discovered the sclera has a mean elevation difference of about 400 microns at this single chord on the same eye and that the shallowest and the deepest semi-meridians were not orthogonal. These data and other results like them support the notion that a preferred periphery of scleral lenses may be rotationally asymmetric.
The Boston Foundation for Sight patented a diagnostic set system that incorporates a similar rotationally asymmetric profile. The potential to use biometric mean data for the peripheral aspect of the posterior surface of lenses while having a single measurement of overall sagittal depth in a single meridian holds promise for technology-driven parameter selection.11
SUMMARY
The body of knowledge about ocular contour combined with the ability to manufacture and measure rotationally asymmetric lenses sets the stage for technology-driven parameter selection as a replacement for diagnostic sets with limited permutations and reliance on practitioner observations—combined with observation-driven calculations—for determining final contact lens parameters.
All required instrumentation is available for technology-driven parameter selection for initial orthokeratology lenses. The present gap in the critical mass of instrumentation required for parameter selection of lenses that extend beyond the limbus is in the availability of instrumentation for measuring ocular contour.
The increased adoption of freeform soft, hybrid, scleral, and component-containing smart lenses is forecast in conjunction with the megatrend of digital health and telemedicine technology that enables remote care and holds the potential for increased efficiency in care delivery, with resultant cost containment and affordability of advanced contact lens care. CLS
References
- Fogt JS. Novel silicone elastomer contact lenses designed for simultaneous viewing of distance and near eye displays. Cont Lens Anterior Eye. 2023 Aug;46:101870.
- Cooley SS, Deffler R, Legerton J, Marsh J, Dougherty BE. Evaluation of a New Device for Distance and Reading Tasks in Low Vision. Invest Ophthalmol Vis Sci. 2021 Jun;62:3567.
- Legerton JA, Liu J, inventors. Geometric Volume Control Corneal Refractive Therapy Contact Lenses. U.S. patent 20230103752. 2023. Available at patents.google.com/patent/US20230103752A1/en?oq=US+patent+20230103752 . Accessed Oct. 3, 2023.
- Holden BA, Mertz GW. Critical oxygen levels to avoid corneal edema for daily and extended wear contact lenses. Invest Ophthalmol Vis Sci. 1984 Oct;25:1161-1167.
- Su B, Yu A, Chung D, Greenstein S, Gelles JD. Success with Soft Contact Lenses after Failure of Rigid Contact Lenses in Keratoconus. Invest Ophthalmol Vis Sci. 2023 Jun;64:3539.
- Hastings GD, Applegate RA, Nguyen LC, Kauffman MJ, Hemmati RT, Marsack JD. Comparison of Wavefront-guided and Best Conventional Scleral Lenses after Habituation in Eyes with Corneal Ectasia. Optom Vis Sci. 2019 Apr;96:238-247.
- Young G. Mathematical model for evaluating soft contact lens fit. Optom Vis Sci. 2014 Jul;91:e167-e176.
- Legerton JA, inventor. Non-deforming contact lens. U.S. patent 20100110382. 2010 May 6. Available at patents.google.com/patent/US20100110382A1/en?oq=US+patent+20100110382 . Accessed Oct. 3, 2023.
- van der Worp E, Lampa M, Kinoshita B, Fujimoto MJ, Coldrick BJ, Caroline P. Variation in sag values in daily disposable, reusable and toric soft contact lenses. Cont Lens Anterior Eye. 2021 Dec;44:101386.
- Yu A, Su B, Jaki N, et al. A Review of Scleral Shape from Scheimpflug Profilometry. Poster presented at the Global Specialty Lens Symposium, Las Vegas. January 2023.
- Carrasquillo KG, Patel C, Remington C, Kwok A, Jacobs DS, inventors. Chiral scleral lenses. U.S. Patent 9874766. 2018 Jan 23. Available at patents.google.com/patent/US9874766B2/en?oq=U.S.+Patent+9874766 . Accessed Oct. 3, 2023.