
The field has moved beyond using only curvature data to guide contact lens design to include data on shape, elevation, sagittal depth, and other indices. Lens options are evolving from stock designs to customized or free-form designs. This article explores the technologies of topography and tomography, and how they may be used to influence the contact lens fitting process.
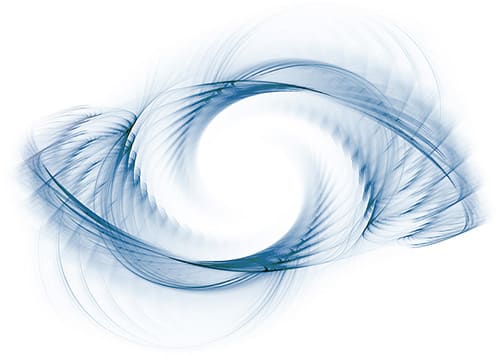
It is difficult to obtain detailed information on the acquisition and utilization of topographers and/or tomographers, and many devices offer multiple other features. A technology purchase survey of 276 optometrists found that 52.8% of existing practices owned a corneal topographer and 17.5% planned to acquire one.1 The number of those owning or planning on acquiring an anterior segment tomographer is more challenging to determine and was not reported as a separate category.
Of the practices participating in the survey, 67.3% reported that they own an optical coherence tomographer (OCT) and 32.3% planned to acquire one.1 Fifty-two percent of those anticipating acquisition planned to buy devices capable of anterior segment OCT tomography, while 48% emphasized posterior segment capabilities.1
CONTRAST OF TECHNOLOGIES
Topography and tomography share some similarities but vary greatly in how they obtain data, the area of coverage of the anterior segment, and their limitations. They are not interchangeable. Topography is the older of the two technologies and can trace its origins back to the 17th century,2 but the modern age began with Placido de Costa’s work in 1880, which relied on the reflection of the mire patterns off the cornea to calculate the surface curvature.3
Steeper surfaces are reflected in closer spacing of the mire rings, while flatter surfaces increase the spacing between the mires. Contemporary devices still apply these same principles with improvements in lighting, video capture, and computerized analysis. The more data points collected, the greater the accuracy.
This led to small-cone devices with shorter working distances and greater coverage of the area of interest, allowing for closer spacing between rings and less extrapolation of the data, although they are somewhat limited when used with deep-set eyes compared to large-cone devices.2 There are a few notable sources of error that are associated with this method.
If the reflection of the mires off the tear film is not clear due to instability, then data gaps result and the calculation of any values derived from these images will be in error. If the corneal surface is irregular, values calculated from a reference axis result in skew errors as well.4
There are also devices that rely on a color-emitting diode, which may avoid the skew errors found with typical Placido disc-based devices.2 These devices may be combined with autorefractors, wavefront refractors, meibography, noninvasive tear breakup times, or other features.
Anterior segment OCT, scanning slit, and Scheimpflug camera tomography devices offer the advantage of obtaining more than surface curvature data, including posterior surface and pachymetry data, while avoiding the errors irregular corneas create for topographers.5 They use either an interferometer (time domain OCT, spectral domain OCT, or swept-source OCT), slit scanning, or Scheimpflug principles, pioneered in 1904, to evaluate the corneal surfaces and may be affected by opacities that interfere with the backscatter of light.6
Various devices offer an expanded range of biometric data, including anterior chamber analysis, axial length, corneal optical densitometry, Hartmann-Shack aberrometry, epithelial thickness mapping, and even noncontact tonometry. These devices measure true corneal power, elevation, and height data rather than assuming a constant relationship between the anterior and posterior corneal surfaces or calculating these values from a reference axis along a meridian.2 They are not affected by tear film stability and are likely more accurate for irregular corneas, although eye movement can influence image quality due to slower image collection speeds.5,7 Scheimpflug devices produce lower resolution images than OCT devices and with less detail of the anterior chamber.8
BIOMETRY AND INDICES
Improving outcomes in anterior segment patient care requires us to make an early and accurate diagnosis, identify progression of corneal disease states, and use data to inform contact lens design choices. Regardless of which device(s) you use, you will be presented with large amounts of data and many ways to display the results. Some are common to all devices and some are unique to a particular device.
Curvature is described numerically as simulated keratometry (simK) values for the flat and steep principal meridians, average K-value, and maximum K-value for both devices. Data are often displayed referenced to the instrument axis as axial (sagittal) maps, which represents the optical characteristics of the corneal surface, facilitating comparison with other eyes.
Tangential (instantaneous) maps present curvature at each of the points measured, making it more useful when evaluating the topographical details of an individual eye. It is particularly useful for following changes over time in progressive ectasias or when performing orthokeratology.
Other useful maps include power, elevation, height, and difference plots.9 The elevation maps provide information necessary to create simulated fluorescein images representing the tear film thickness between the posterior lens surface and the anterior corneal surface. Remember, tomographers, including rotating slit and Scheimpflug devices, provide more precise data, as the values are directly measured and not calculated as in topography.
It is important to recognize that if the scale used on a given map is insufficiently fine in increment (i.e., 0.25D versus 1.00D), you will not be able to separate out subtle changes and they will be obscured by the largest variations in curvature. Normal values for a regular cornea will range from 42.00D to 45.00D,10 and under the modified Rabinowitz-McDonnell method, central powers > 47.2D are considered suspect for an ectasia.11
Shape descriptors include e-value (eccentricity), Q-value (asphericity), and p-value. These values are based on conic sections, each with advantages and disadvantages. Eccentricity represents the amount of corneal flattening. Values will fall between 0 (circle) and 1 (an ellipse) with a reported average of 0.43 (range 0.40 to 0.57 for normal corneas). It does not tell you if the shape is prolate or oblate. This is an advantage of Q- and p-values, where prolate is a negative value and oblate is a positive value. They are related where Q = p – 1 and p = 1 – e2. For Q-values, 0 is a sphere and for p-values, 0 is an ellipse.9 Typical values for asphericity Q-values have racial variations but are in the 0.20 to 0.26 range.12
There are a host of indices and displays available to assist in assessing irregular corneas. Steepest radial axis (SRAX) asymmetry (> 21° is abnormal) compares symmetry across halves of a meridian;9 inferior-superior (I-S) asymmetry (1.4D to 1.9D moderate suspect, > 1.9D highly suspect) compares across five points in the superior cornea to inferior;9 surface asymmetry index (SAI) compares a centrally weighted average corneal power to the power and location of the flattest and steepest corneal meridians;13 surface regularity index (SRI) within 4.0mm pupil (< 1.0 is normal)14 and correlates well with visual acuity are among the most common ones.
It becomes more complicated when looking at indicators of keratoconus on OCT and Scheimpflug devices, where there are many more derived values, including validated cut-off values for anterior (≥ 15µm for suspect, ≥ 19µm for keratoconus)15 and posterior (≥ 35µm for suspect, ≥ 51µm for keratoconus) corneal elevations.15,16 There are many more that are specific to particular devices (e.g., Enhanced Belin/Ambrosio display), but this is a fair representation of the most common ones.
Many devices possess the ability to simulate fluorescein patterns, facilitate free-form design work, and create difference plots assessing change across visits. The ability to simulate fluorescein patterns based on corneal elevation data to predict a fitting relationship is particularly helpful in initial lens selection and parameter changes with corneal GP (for both regular and irregular corneas) as well as for orthokeratology fits.
Some devices allow the practitioner to select soft lens parameters from manufacturer files downloaded into the device. Devices are increasingly able to upload files directly to the laboratory for consultation or fabrication of corneal GP, orthokeratology, and scleral designs. Advanced fitters may take advantage of the ability to directly export a patient file into design software, providing practitioners with complete control over the design process prior to transmission to the laboratory.
The ability to compare various biometric readings across visits for change allows practitioners to adjust lens parameters prior to adverse events or to make timely referrals for surgical interventions by considering multiple biometric parameters with confidence intervals compared to normative or keratoconus patient base (e.g., ABCD progression analysis).
APPLICATION TO FITTING
Trial lens fitting remains the mainstay of clinical soft contact lens fitting on regular corneas.17 The utility of the additional information provided by topographers and tomographers increases when fitting patients who fall outside the bell-shaped curves or nomograms of typical patients. This includes regular corneas with large horizontal visible iris diameters (HVIDs), keratometry values less than 42.00D or greater than 45.00D,10 and the constellation of irregular corneas created by pathologies or surgical interventions.
These devices can provide HVID, white-to-white (WTW) values, and the ability to use biometric calipers to determine the approximate corneal dimensions. Normal values vary by device but range between 11.5mm and 12.5mm, with microcorneas defined as < 10.0mm to 11.0mm and macro-corneas > 12.0mm.18
Several studies demonstrate that central keratometry values are a poor predictor of overall soft lens fit and that factors affecting sagittal height of the cornea (i.e. corneal diameter, shape, and asphericity) may be better predictors.17,19-21 These values are readily available in most topographers and tomographers, although topographers are more limited in their ability to look much beyond a 10mm chord.
The premise is that matching these factors to a given ocular surface may improve the overall fit and comfort.22 If we know both the ocular sagitta and the lens sagitta, we can be more intentional in changing parameters to address a loose- or tight-fitting soft lens. Fortunately, there are some studies that may provide us with the guidance we need to reduce the number of trial fits on these more complicated soft lens fits on regular corneas.22,23
Corneal GP lenses vary from small to intralimbal diameters. Achieving an optimal GP lens fit is multifactorial, with both anatomical and lens considerations. These include lid aperture size, lid-lens position, corneal diameter, corneal shape, corneal sagitta, tear meniscus height, contact lens center of gravity, back optic zone radius, overall diameter, and the interactions between all these factors and more.24,25
Early studies of topography software used to facilitate GP contact lens fitting found they were generally accurate and improved fitting efficiency, and that practitioner guidance was needed to optimize fitting.26 Studies using contemporary devices that compare empirical and trial lens fitting are lacking for regular corneas. Much more information is available on the application of this technology to fitting irregular corneas. More on that later.
One study looked at orthokeratology (a subset of corneal GP lenses) used on regular corneas. This retrospective study, using topography, found that the difference in elevation maps in the central 8mm chord correlated well with the need for dual-axis lens designs but not with flat K, flat e, or steep e.27 The authors provide a linear regression formula that allows a practitioner to predict how much toricity is needed in the lens to increase the likelihood of centration and improved effect, particularly when managing limbus-to-limbus astigmatism.
One proprietary design begins offering a dual-axis design when the difference at the 8mm chord is 30µm in 30µm increments. Many of these devices allow you to upload the data directly to the laboratory for manufacture and allow them to incorporate the toric zones into their novel designs as needed.
We can use the data from these devices to enhance our fitting of hybrid contact lenses. One study looked at subjects (N = 18 eyes) who had regular corneal astigmatism greater than 3.00D (range 3.44D to 6.47D), included after obtaining data via a Scheimpflug device, who were then fit with a spherical hybrid contact lens approximately 1.00D steeper than flat K.28 The authors found that this lens design provided “optimal visual function with high comfort, and patient satisfaction”28 for regular astigmats in these higher cylinder powers.
Fitting scleral contact lenses requires an understanding of the symmetry of the scleral profiles, which are only spherical in 5.7% of the time.29 Fourier domain scleral profilometry is the gold standard for detailing the scleral profile. Scleral profilometry is available on a Scheimpflug device, whereas topographers generally lack the ability to sufficiently cover the scleral profile in appropriate detail. The information obtained by Scheimpflug devices is not interchangeable with Fourier domain profilometry, but still useful.30,31 Software upgrades can suggest starting sagittal depths in relationship to a given overall lens diameter.
Elevation differences can be measured by both topographers and tomographers. Two studies presented as posters at the Global Specialty Lens Symposium (GSLS) suggested that an elevation difference of < 350µm along the meridian of greatest change has been shown to be associated with an 88% success rate with corneal GP versus scleral contact lenses.32,33 Although we see increasing use of scleral lens designs for regular corneas for high astigmats, multifocal, and patients who have ocular surface disease, their greatest utility remains with irregular corneas.
Up to this point, only regular corneas have been considered. Topography and tomography devices reach their zenith in providing useful fitting information for irregular corneas. Clinicians are trained to understand the range of normal presentations of the ocular anatomy prior to learning those of various disease states. If you master the use of these devices on regular corneas, you will recognize pathological states more quickly and improve your fitting efficiencies.
Some of the more common applications are screening for or monitoring progression of keratoconus, following corneal graft health, pre-/postoperative care of refractive surgery cases, and assessing higher-order aberrations (HOAs). Studies demonstrate that wave error-correcting scleral lens designs may help normalize these HOAs for keratoconus patients, although much work remains to improve the accuracy of candidate selection and the ability to predict outcomes.34,25 Tear film stability, pupil size, accommodation, and decentration are a few of the confounding variables that make these designs more challenging.
It is important to recognize that the less severe the corneal irregularity, the more contact lens options will be able to provide functional, healthy vision. The more severe the curvature, the greater the need to bridge the apex and improve centration with a hybrid/piggyback or scleral contact lens design. The more decentered the apex, the larger the lens diameter required, including intralimbal, hybrids/piggyback, and scleral designs. A common fitting approach is to pursue the simplest, least expensive, and easiest-to-handle lens options first.
• CASE #1 KERATOCONUS
A 33-year-old African American female who had keratoconus became intolerant of the discomfort of her corneal GP design. Key considerations are that this patient has one radius of curvature greater than 51.21D, corneal astigmatism of 5.72D, a prolate Q-value of –0.11, an inferiorly decentered apex, and a WTW value of 13.16mm. Arguably, an Amsler-Krumeich Grade II out of IV severity exists (Figure 1).36 Possible contact lens options include a specialty soft lens for keratoconus, refit into a corneal GP design, hybrid/piggyback to ensure centration, or a scleral contact lens. The patient was averse to trying another GP lens design and was refit into a specialty soft lens for keratoconus, which improved comfort and slightly improved her best-corrected visual acuity (BVCA) to 20/25.
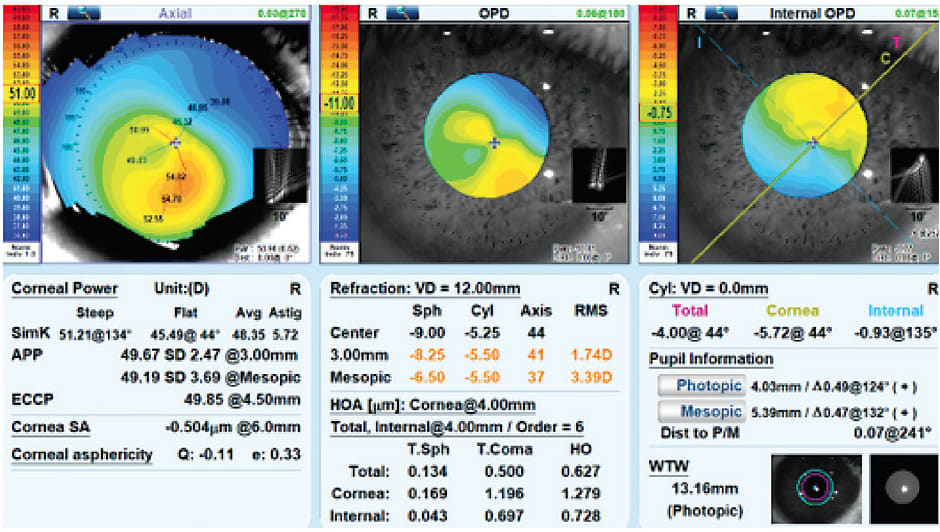
• CASE #2 PENETRATING KERATOPLASTY
A 33-year-old African American male with a history of keratoconus and bilateral penetrating keratoplasties OD (2006) and OS (2011) was referred by a local corneal specialist for a scleral contact lens fitting. Key points to consider are that the graft is tilted superior nasal with a temporal island, 8.00D of irregular astigmatism, and generally oblate shape (Figure 2). The best option for this patient due to the oblate shape, tilt, and irregularities is a scleral contact lens. He was successfully fit bilaterally, obtaining a BCVA of 20/25.

FIGURE 2. Penetrating keratoplasty right eye. Photos courtesy of Daniel G. Fuller, OD
• CASE #3 ORTHOKERATOLOGY
A 15-year-old-East Asian male was referred by an ophthalmologist for myopia management after not responding well to myopia control efforts on low-dose atropine. Parents and patient understood that efforts would be off-label, but requested anything that could be done be pursued. Baseline cycloplegic refraction was –7.75 –0.50 x 035 (20/20) OD (Figure 3). There is some evidence suggesting that a combined approach with low-dose atropine and orthokeratology may offer some benefit.37 However, this patient was outside of the inclusion criteria of the cohorts studied based on his refractive error, age, and available U.S. Food and Drug Administration (FDA)-approved options.

FIGURE 3. Orthokeratology fit right eye. A) Baseline topography; B) Approximately four months later; and C) Comparison map of corneal power from start to finish. Photos courtesy of Daniel G. Fuller, OD
Even for myopia management, there are challenges in fitting an orthokeratology option. The corneal elevation differences in the central 8mm were approximately 35µm, requiring a toric design, and the refractive error exceeded the upper limits of most approved stock designs. A more customized or free-form toric design in a large overall diameter with a smaller treatment zone was required. Note the decentration of the treatment zone relative to the pupil center may cause complaints of glare and halos with scotopic pupil dilation or use of low-dose atropine. HOAs from uncorrected asymmetric elevation differences may also contribute.
The patient was refit into a free-from toric design and low-dose atropine was discontinued. The treatment effects improved from the original spherical lens that was dispensed and the dysphotopsia abated. The final manifest refraction is plano (20/15).
CONCLUSION
As topography and tomography devices become more affordable, our ability to integrate a deeper understanding of the role of curvature, shape, and peripheral ocular surface profiles into contact lens designs has increased. Understanding how to use these devices to best effect allows practitioners to exploit the nuances of individual contact lens designs, offer free-form designs, and avoid falling into the trap of a one-size-fits-all approach. Patients benefit most from early detection of disease processes and the availability of multiple contact lens options. CLS
References
- Manthorp C. New Technology Purchases: How and Why ODs Buy. Rev Optom. 2022 Sept 15;159:38-42.
- Kanclerz P, Khoramnia R, Wang X. Current Developments in Corneal Topography and Tomography. Diagnostics (Basel). 2021 Aug 13;11:1466.
- Plácido A. Novo instrumento de exploração da córnea. Periódico d’Oftalmológica Practica (Lisboa). 1880;5:27-30.
- Klein SA. Axial curvature and the skew ray error in corneal topography. Optom Vis Sci. 1997 Nov;74:931-944.
- Martin R. Cornea and anterior eye assessment with placido-disc keratoscopy, slit scanning evaluation topography and scheimpflug imaging tomography. Indian J Ophthalmol. 2018 Mar;66:360-366.
- Faria-Correia F, Ambrósio F. Clinical applications of the Scheimpflug principle in Ophthalmology. Rev Bras Oftalmol. 2016 Apr;75:160-165.
- Wegener A, Laser-Junga H. Photography of the anterior eye segment according to Scheimpflug’s principle: options and limitations - a review. Clin Exp Ophthalmol. 2009 Jan;37:144-154.
- Savini G, Schiano-Lomoriello D, Hoffer KJ. Repeatability of automatic measurements by a new anterior segment optical coherence tomographer combined with Placido topography and agreement with 2 Scheimpflug cameras. J Cataract Refract Surg. 2018 Apr;44:471-478.
- van der Worp E, de Brabander J, Jongsman F. Corneal topography. In: Bennett ES, Henry VA, eds. Clinical Manual of Contact Lenses. Vol 4th Ed. Philadelphia: Lippincott Williams & Wilkins; 2014:54-88.
- Alshehri O, Abdelaal AM, Abudawood G, et al. Normative Values for Corneal Tomography and Comparison of Both Eyes in Young Saudi Males with 20/20 Vision Using Pentacam-HR Scheimpflug Imaging. Clin Ophthalmol. 2022 Aug 13;16:2631-2637.
- Maeda N, Klyce SD, Smolek MK. Comparison of methods for detecting keratoconus using videokeratography. Arch Ophthalmol. 1995 Jul;113:870-874.
- Fuller DG, Alperin D. Variations in corneal asphericity (Q value) between African-Americans and whites. Optom Vis Sci. 2013 Jul;90:667-673.
- Dingeldein SA, Klyce SD, Wilson SE. Quantitative descriptors of corneal shape derived from computer-assisted analysis of photokeratographs. Refract Corneal Surg. 1989 Nov-Dec;5:372-378.
- Wilson SE, Klyce SD. Quantitative descriptors of corneal topography. A clinical study. Arch Ophthalmol. 1991 Mar;109:349-353.
- Jafarinasab MR, Shirzadeh E, Feizi S, Karimian F, Akaberi A, Hasanpour H. Sensitivity and specificity of posterior and anterior corneal elevation measured by Orbscan in diagnosis of clinical and subclinical keratoconus. J Ophthalmic Vis Res. 2015 Jan-Mar;10:10-15.
- de Sanctis U, Loiacono C, Richiardi L, Turco D, Mutani B, Grignolo FM. Sensitivity and specificity of posterior corneal elevation measured by Pentacam in discriminating keratoconus/subclinical keratoconus. Ophthalmology. 2008 Sep;115:1534-1139.
- Young G, Schnider C, Hunt C, Efron S. Corneal topography and soft contact lens fit. Optom Vis Sci. 2010 May;87:358-366.
- Gharaee H, Abrishami M, Shafiee M, Ehsaei A. White-to-white corneal diameter: normal values in healthy Iranian population obtained with the Orbscan II. Int J Ophthalmol. 2014 Apr 18;7:309-312.
- Hall LA, Young G, Wolffsohn JS, Riley C. The influence of corneoscleral topography on soft contact lens fit. Invest Ophthalmol Vis Sci. 2011 Aug 29;52:6801-6806.
- Young G. Mathematical model for evaluating soft contact lens fit. Optom Vis Sci. 2014 Jul;91:e167-e176.
- Young G, Hall L, Sulley A, Osborn-Lorenz K, Wolffsohn JS. Inter-relationship of Soft Contact Lens Diameter, Base Curve Radius, and Fit. Optom Vis Sci. 2017 Apr;94:458-465.
- Giovanzana S, Talu S, Nicoara SD. Sagittal height differences of disposable soft contact lenses. Int Ophthalmol. 2020 Feb;40:459-465.
- van der Worp E, Lampa M, Kinoshita B, Fujimoto MJ, Coldrick BJ, Caroline P. Variation in sag values in daily disposable, reusable and toric soft contact lenses. Cont Lens Anterior Eye. 2021 Dec;44:101386.
- Carney LG, Mainstone JC, Carkeet A, Quinn TG, Hill RM. The influence of center of gravity and lens mass on rigid lens dynamics. CLAO J. 1996 Jul;22:195-204.
- Cardona G, Isern R. Topography-based RGP lens fitting in normal corneas: the relevance of eyelid and tear film attributes. Eye Contact Lens. 2011 Nov;37:359-364.
- Szczotka LB. Clinical evaluation of a topographically based contact lens fitting software. Optom Vis Sci. 1997 Jan;74:14-19.
- Ling JJ, Mian SI, Stein JD, Rahman M, Poliskey J, Woodward MA. Impact of Scleral Contact Lens Use on the Rate of Corneal Transplantation for Keratoconus. Cornea. 2021 Jan;40:39-42.
- Abou Samra WA, El-Emam DS, Kasem MA. Clinical Performance of a Spherical Hybrid Lens Design in High Regular Astigmatism. Eye Contact Lens. 2018 Sep;44 Suppl 1:S66-S70.
- DeNaeyer G, Sanders DR, van der Worp E, Jedlicka J, Michaud L, Morrison S. Qualitative Assessment of Scleral Shape Patterns Using a New Wide Field Ocular Surface Elevation Topographer: The SSSG Study. JCLRS. 2017 Nov;1:12-22.
- Bataille L, Molina-Martin A, Piñero DP. Comparative Analysis of Two Clinical Diagnostic Methods of the Corneoscleral Geometry. Eye Contact Lens. 2021 Oct;47:546-551.
- Bandlitz S, Esper P, Stein M, Dautzenberg T, Wolffsohn JS. Corneoscleral Topography Measured with Fourier-based Profilometry and Scheimpflug Imaging. Optom Vis Sci. 2020 Sep;97:766-774.
- Kojima R, Caroline P, Lampa M, Fujimoto M, André M, Nguyen C. To Corneal GP or Not to Corneal GP, That Is the Question? Poster presented at the 2023 Global Specialty Lens Symposium, Las Vegas. January 2023.
- Zheng F, Caroline P, Kojima R, Kinoshita B, André M, Lampa M. Corneal Elevation Differences and the Initial Selection of Corneal and Scleral Contact Lens. Poster presented at the 2015 Global Specialty Lens Symposium, Las Vegas. January 2015.
- Sabesan R, Johns L, Tomashevskaya O, Jacobs DS, Rosenthal P, Yoon G. Wavefront-guided scleral lens prosthetic device for keratoconus. Optom Vis Sci. 2013 Apr;90:314-323.
- Marsack JD, Ravikumar A, Nguyen C, et al. Wavefront-guided scleral lens correction in keratoconus. Optom Vis Sci. 2014 Oct;91:1221-1230.
- Krumeich JH, Daniel J, Knulle A. Live-epikeratophakia for keratoconus. J Cataract Refract Surg. 1998 Apr;24:456-463.
- Kinoshita N, Konno Y, Hamada N, et al. Efficacy of combined orthokeratology and 0.01% atropine solution for slowing axial elongation in children with myopia: a 2-year randomised trial. Sci Rep. 2020 Jul 29;10:12750.