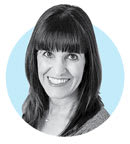
THE LOSS OF TEAR FILM homeostasis has been recognized by the Tear Film & Ocular Surface Society Dry Eye Workshop II (TFOS DEWS II) and tear hyperosmolarity has been established as a core mechanism of dry eye disease (DED). Hyperosmolar tears have been reported to disrupt the balance of the ocular surface, mainly by influencing ocular inflammation. This occurs through the corneal epithelial cells’ release of inflammatory cytokines, chemokines, and matrix metalloproteinases (MMP) like MMP-9 from the epithelial cells (Li et al, 2006; Panigrahi et al, 2019; Pflugfelder et al, 2008; Igarashi et al, 2014; and others. Full list available below).
Several studies have shown that when human corneal epithelial cells are subjected to hyperosmolar and desiccation stress, they exhibit notable responses in inflammatory cytokine interleukin (IL)-6, chemokine (IL-8), and monocyte chemoattractant protein-1 (MCP-1) production (Cavet et al, 2010; Higuchi et al, 2011). Thus, this study measured these pro-inflammatory mediators.
Wearing contact lenses (CLs) disrupts tear film homeostasis, the natural balance of the ocular surface environment, by augmenting water evaporation and osmolarity of the tears. Preserving homeostasis of the ocular surface is essential for both CL wearers and manufacturers (VanDerMeid et al, 2024). Recognizing that ensuring ocular homeostasis while wearing CLs holds promise for enhancing both comfort and vision, manufacturers have created various technologies to support homeostasis (Efron et al, 2013). One option is to increase lens water retention during wear (Schafer et al, 2018; Shafer et al, 2021). Another option is to infuse beneficial ingredients into the lens (Reindel et al, 2023).
The literature demonstrates wide variations in pH, osmolarity, and surface tension among commercial CL blister package solutions. There is variability in contact angle among different combinations of lenses and solutions, indicative of the surfactants in the solutions and the specific characteristics of the lenses (Menzies et al, 2010; Menzies et al, 2011).
A study evaluated the metabolic movement and inflammatory responses of a transformed human corneal epithelial cell (THCEpiC) line in a hyperosmotic environment in the presence of various solutions used in CL packaging (VanDerMeid et al, 2024). Seven silicone hydrogel daily replacement CL blister packaging solutions were sampled, concentrated at 25%, and made hyperosmolar (400 mOsm/kg) with sodium chloride. THCEpiCs were exposed to each solution for 24 hours, after which cell culture supernatants were gathered. An alamarBlue assay was used to evaluate THCEpiC metabolic activity. Specific immunosorbent assays that were enzyme-linked were employed to quantify concentrations in cell culture supernatants of IL-6, IL-8, and MCP-1.
Under hyperosmolar conditions, the metabolic activity of THCEpiC decreased with two solutions: somofilcon A and senofilcon A (p = 0.04 and 0.004, respectively), while IL-6 concentrations increased with delefilcon A, somofilcon A, narafilcon A, and senofilcon A solutions (all p ≤ 0.001), and IL-8 concentrations increased with all solutions except kalifilcon A (all p ≤ 0.03). The concentrations of MCP-1 increased with delefilcon A, verofilcon A, somofilcon A, and stenfilcon A solutions (all p < 0.0001).
In this investigation, all assessed lenses contained wetting agents such as humectant and/or surfactant wetting agents in the solution. Additionally, kalifilcon A incorporates osmoprotectants utilized in other ocular solutions, along with potassium electrolytes (Baudouin et al, 2013; Jones et al, 2017).
CL packaging solutions vary in their ability to prevent inflammation of the epithelium. In vitro, THCEpiC cells exhibited a reduced inflammatory response when exposed to a CL packaging solution containing osmoprotectants compared to solutions lacking osmoprotectants, particularly in relatively moderate hyperosmolar conditions.
The corneal epithelium may be protected from inflammation under hyperosmotic conditions that occur in CL-related DED by including osmoprotectants in CL packaging solutions. Components such as surfactants and potassium electrolytes may help preserve ocular surface homeostasis, yet their full potential remains to be determined.
References
1. Li DQ, Luo L, Chen Z, Kim HS, Song XJ, Pflugfelder SC. JNK and ERK MAP kinases mediate induction of IL-1beta, TNF-alpha and IL-8 following hyperosmolar stress in human limbal epithelial cells. Exp Eye Res. 2006 Apr;82:588-596.
2. Panigrahi T, Shivakumar S, Shetty R, Stern ME. Trehalose augments autophagy to mitigate stress induced inflammation in human corneal cells. Ocul Surf. 2019 Oct;17:699-713.
3. Pflugfelder SC, de Paiva CS, Li DQ, et al. Epithelial-immune cell interaction in dry eye. Cornea. 2008 Sep;27 Suppl 1:S9-S11.
4. Igarashi T, Fujimoto C, Suzuki H, et al. Short-time exposure of hyperosmolarity triggers interleukin-6 expression in corneal epithelial cells. Cornea. 2014 Dec;33:1342-1347.
5. Liu Z, Chen D, Chen X, et al. Autophagy activation protects ocular surface from inflammation in a dry eye model in vitro. Int J Mol Sci. 2020 Nov 26;21:8966.
6. Cavet ME, Harrington KL, Ward KW, Zhang JZ. Mapracorat, a novel selective glucocorticoid receptor agonist, inhibits hyperosmolar-induced cytokine release and MAPK pathways in human corneal epithelial cells. Mol Vis. 2010 Sep 2;16:1791-1800.
7. Higuchi A, Kawakita T, Tsubota K. IL-6 induction in desiccated corneal epithelium in vitro and in vivo. Mol Vis. 2011;17:2400-2406.
8. VanDerMeid KR, Byrnes MG, Millard K, Scheuer CA, Phatak NR, Reindel W. Comparative Analysis of the Osmoprotective Effects of Daily Disposable Contact Lens Packaging Solutions on Human Corneal Epithelial Cells. Clin Ophthalmol. 2024 Jan;18:247-258.
9. Efron N, Brennan NA, Bright FV, et al. Contact lens care and ocular surface homeostasis. Cont Lens Anterior Eye. 2013 Jan 15;36(Suppl 1):S9-S13.
10. Schafer J, Reindel W, Steffen R, Mosehauer G, Chinn J. Use of a novel extended blink test to evaluate the performance of two polyvinylpyrrolidone-containing, silicone hydrogel contact lenses. Clin Ophthalmol. 2018 May 3;12:819-825.
11. Schafer J, Steffen R, Mosehauer G, et al. Evaluation of the stability of surface water characteristics of contact lenses using refractive index shifts after wear of daily disposable lenses. Abstract presented at the Global Specialty Lens Symposium. 2021 Jan. Virtual.
12. Reindel W, Steffen R, Mosehauer G, et al. Performance of a silicone hydrogel daily disposable contact lens among wearers with lens-related dryness. Open J Ophthalmol. 2023 Mar 31;17:e187436412303021.
13. Menzies KL, Rogers R, Jones L. In vitro contact angle analysis and physical properties of blister pack solutions of daily disposable contact lenses. Eye Contact Lens. 2010 Jan;36:10-18.
14. Menzies KL, Jones L. In vitro analysis of the physical properties of contact lens blister pack solutions. Optom Vis Sci. 2011 Apr;88:493-501.
15. Baudouin C, Aragona P, Messmer EM, et al. Role of hyperosmolarity in the pathogenesis and management of dry eye disease: proceedings of the OCEAN group meeting. Ocul Surf. 2013 Oct;11:246-258.
Jones L, Downie LE, Korb D, et al. TFOS DEWS II management and therapy report. Ocul Surf. 2017 Jul;15:575-628.