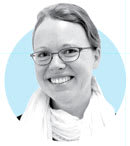
SMART CONTACT LENSES (SCLs) promise exciting new health indications beyond vision correction, with potential features including noninvasive, continuous monitoring of various health conditions, real-time data transfer, and drug release upon threshold crossing. However, for continuous performance and particular access to the measurements, the lenses must either use optical/colorimetric-based systems or have a power supply. The power source can be integrated into the lens, external, or a combination thereof.
IN-VIVO POWERING
Power systems integrated into lenses must have a small footprint, be flexible and safe, and not obstruct the wearer’s vision. Biofuel cells, solar cells, and flexible batteries are the most explored options. Biofuel cells use tear film elements, such as ascorbate and oxygen (Falk et al, 2013), lactate (Xiao et al, 2018), and glucose (Kang et al, 2022) to produce energy. The average maximum output of these power sources is approximately 0.5V, which may be sufficient for simple SCLs but may not provide enough power for multisensing SCLs.
The use of solar cells in SCLs is still in early development; recently, however, optimized organic solar cells demonstrated an output of 0.6V in indoor lighting conditions, sufficient to power sensors for glucose and calcium measurements and transfer the measurements to a portable device via near field communication (Lin et al, 2022). Others combined flexible solar cells and a magnesium-oxygen harvester activated by the tear film through blinking (Pourshaban et al, 2024). As the eyelid travels across the harvester, spreading the tear film, tear film electrolytes react with the electrodes, leading to the creation of energy due to oxidation, with a DC output of 3.3V with different amounts of power.
Solid-state batteries have the advantage of a continuous power supply. In 2018, a flexible, thin solid-state battery was developedfor integration into SCLs, with an output of 3.0V (Lee et al, 2018). However, lens thicknesses typically average 500µm to 1mm, leading to comfort issues. Additionally, safety concerns arise around leakage of toxic and flammable material from these batteries, thereby requiring more biocompatible solutions (Mirzajani et al, 2022). To overcome this issue, a flexible battery that uses the tear film as an electrolyte was developed, which had an output of 0.2V to 0.9V, sufficient for simple SCLs (Yun et al, 2021).
EXTERNAL POWERING
External powering is required when the SCL does not contain an autonomous power source and is typically achieved through wireless power transmission. Power is transferred through inductive coupling between a transmitter antenna (powered by a battery or other power source) and a receiver antenna contained within the SCL. The best-known system is likely the U.S. Food and Drug Administration (FDA)-approved lens for intraocular pressure measurement, in which the transmitting antenna is a ring attached to the skin around the orbit and the receiver antenna is embedded in the SCL (Dunbar et al, 2017).
Other methods include integrating the transmitting antenna into glasses (Takamatsu et al, 2019) or using a smartphone (Park et al, 2024). While this system requires limited elements within the SCL, proximity between the transmitting and receiving antenna is required; in systems using a smartphone, power transfer is only possible when the phone is held close to the eye. Additionally, the smaller the antenna, the higher the induced heat, which may negatively affect the eye.
FUTURE OUTLOOK
The field of SCLs is rapidly advancing. Various power systems are being explored, the goal being to power SCLs that allow for continuous monitoring and drug release without the need to wear glasses or wired batteries or having to hold a smartphone close to the eye, thereby permitting the concealed detection, monitoring, and treatment of certain health conditions.
REFERENCES
1. Dunbar GE, Shen BY, Aref AA. The Sensimed Triggerfish contact lens sensor: efficacy, safety, and patient perspectives. Clin Ophthalmol. 2017 May 8;11:875-882.
2. Falk M, Andoralov V, Silow M, Toscano MD, Shleev S. Miniature biofuel cell as a potential power source for glucose-sensing contact lenses. Anal Chem. 2013 Jul 2;85:6342-6348.
3. Kang D, Lee JI, Maeng B, et al. Safe, Durable, and Sustainable Self-Powered Smart Contact Lenses. ACS Nano. 2022 Oct 25;16:15827-15836.
4. Lee H, Kim S, Kim K-B, Choi J-W. Scalable fabrication of flexible thin-film batteries for smart lens applications. Nano Energy. 2018 Nov;53:225-231.
5. Lin B, Wang M, Zhao C, et al. Flexible organic integrated electronics for self-powered multiplexed ocular monitoring. npj Flexible Electronics. 2022 Aug 25;6:77.
6. Mirzajani H, Mirlou F, Istif E, Singh R, Beker L. Powering smart contact lenses for continuous health monitoring: Recent advancements and future challenges. Biosens Bioelectron. 2022 Feb 1;197:113761.
7. Park W, Seo H, Kim J, et al. In-depth correlation analysis between tear glucose and blood glucose using a wireless smart contact lens. Nat Commun. 2024 Apr 2;15:2828.
8. Pourshaban E, Karkhanis MU, Deshpande A, et al. Power Scavenging Microsystem for Smart Contact Lenses. Small. 2024 Aug;30:e2401068.
9. Takamatsu T, Chen Y, Yoshimasu T, Nishizawa M, Miyake T. Highly efficient, flexible wireless‐powered circuit printed on a moist, soft contact lens. Adv Mat Tech. 2019 Mar 12;4:1800671.
10. Xiao X, Siepenkoetter T, Conghaile PÓ, Leech D, Magner E. Nanoporous gold-based biofuel cells on contact lenses. ACS Appl Mater Interfaces. 2018 Feb 28;10:7107-7116.
11. Yun J, Zeng Y, Kim M, et al. Tear-Based Aqueous Batteries for Smart Contact Lenses Enabled by Prussian Blue Analogue Nanocomposites. Nano Lett. 2021 Feb 24;21:1659-1665.