CONTACT LENSES (CLs) have emerged as powerful tools capable of addressing challenges beyond vision correction.1 Advances in biomaterials, sensor technology, and nanotechnology are driving the development of CLs for diagnosing systemic and ocular diseases, delivering topical drugs, and enabling novel imaging applications.2,3 This article explores the key advancements shaping the future of CLs, focusing on their roles in diagnosing disease, delivering drugs, and providing cutting-edge imaging and augmented reality (AR) solutions, providing insight into a future in which CLs transcend their original purpose.
Much of the technology behind the development of these so-called “smart” CL platforms is driven by the rapid developments in the wearable biosensor market.4,5 The global market for wearable health devices, predicted to reach $30 billion by 2026, underscores the demand for such innovations. However, challenges remain in miniaturizing electronics, ensuring biocompatibility, and maintaining power efficiency.
Conventional batteries cannot be integrated with a smart CL, as those batteries are large and inflexible, produce heat, have a limited lifespan, and use toxic electrolytes.6,7 One intriguing powering option is a technology in which a glucose-based coating reacts with the Na+ and Cl- ions in the tear film surrounding it to generate electricity and power a circuit.8
CLs for Systemic Disease Diagnosis
Advances in biosensing technology have enabled the integration of diagnostic capabilities into CLs.1 They are emerging as a promising platform for noninvasive biosensing of tear fluid biomarkers, including glucose, lactate, matrix metalloproteinases, and cancer.9,10
The detection of glucose in the tear film to diagnose diabetes has been a focus for several research groups, and previous studies have shown that there is a strong correlation between tear and blood glucose levels (R² > 0.8),11 offering a noninvasive alternative to traditional blood-based measurements. Another promising biomarker is Substance P, which correlates with diabetic peripheral neuropathy. By analyzing its concentration in tears, these lenses may help identify the early-stage complications of diabetes.12
Ocular Disease Diagnosis and Monitoring
Smart CLs equipped with continuous monitoring systems are transforming the management of ocular diseases. Diagnosis of glaucoma is a primary focus. A commercially available CL, embedded with a strain gauge, provides 24-hour intraocular pressure (IOP) monitoring by measuring corneal curvature changes (Figure 1).13-15 Such data enable early detection and personalized treatment.
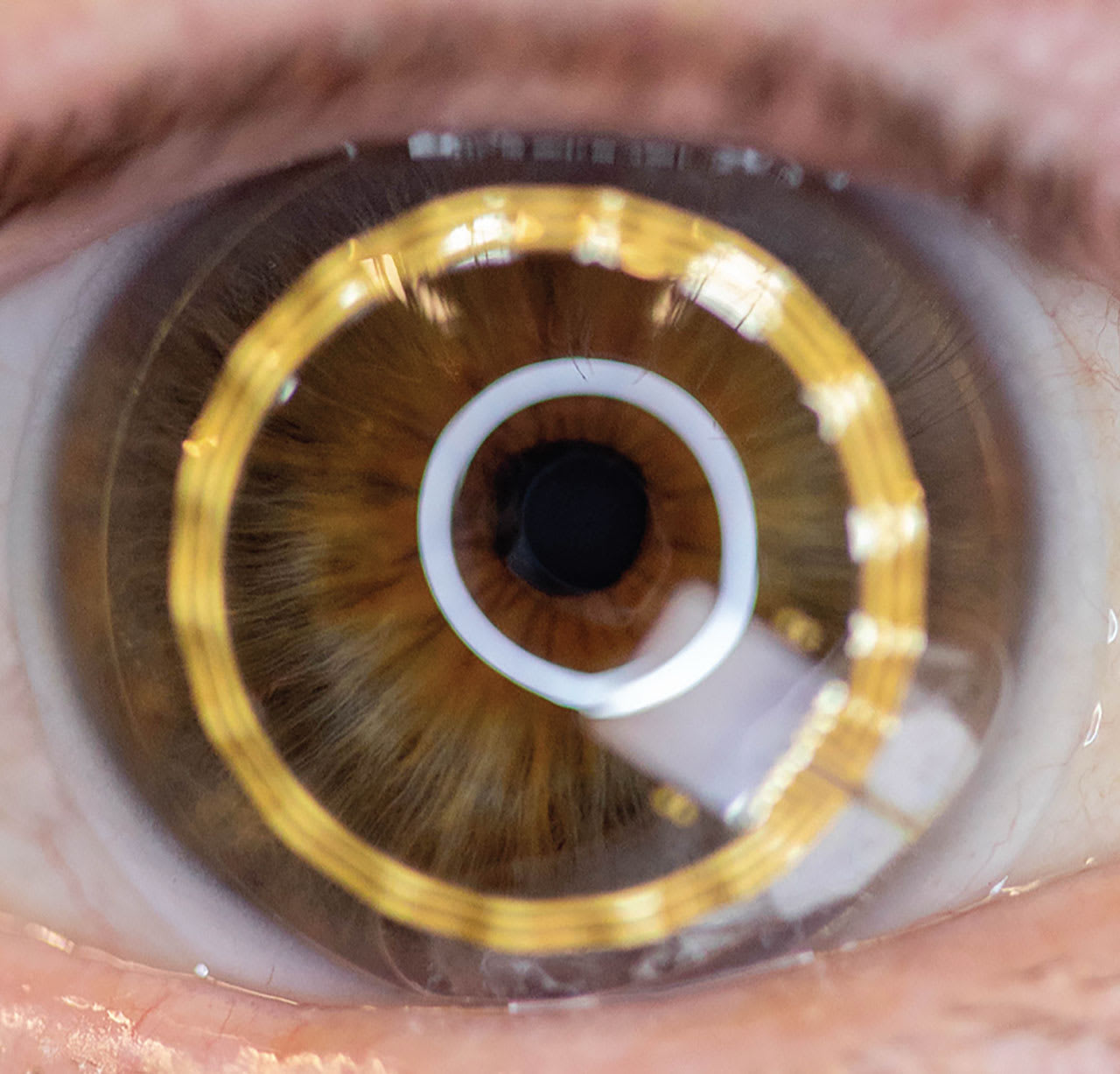
Smart CLs are also being developed to monitor dry eye disease (DED) using biomarkers, including tear osmolarity and inflammatory cytokines (eg, TNF-α, IL-6).16 Additional capabilities, such as corneal temperature measurement and integrated pulse oximetry, promise to enhance the detection and management of a wide variety of ocular conditions.1,17,18
Drug Delivery via CLs
Topical eye drops dominate ocular drug delivery but suffer from poor bioavailability, with less than 5% of the drug instilled reaching the target tissue.19 CLs soaked or embedded with drugs offer a sustained-release mechanism, improving therapeutic efficacy.1,20,21
While commercially available CL materials can uptake and release topical pharmaceutical agents, their release rate is too rapid to make them a viable option for extended drug delivery.1,22,23 Methods such as molecular imprinting, nanoparticles, impregnated polymer films, vitamin E diffusion barriers, and surface printing have been developed to control drug release from lenses.1,24-26 Potential applications include short-term therapies for microbial keratitis and corneal abrasions and longer-term treatments for glaucoma and ocular allergies.1,27-29 Scleral lenses with posterior drug reservoirs to deliver various drugs, including bevacizumab and cyclosporine, further expand their therapeutic potential in diseased eyes that have irregular corneas.30-32
Despite promising results, commercialization faces hurdles, including ensuring consistent drug release profiles and overcoming various regulatory challenges.1,33,34
3D Printing and CLs
3D printing is transforming the development of unique CLs by enabling precise customization, rapid prototyping, and advanced material applications.35,36 Researchers are utilizing biocompatible materials and high-resolution printing techniques to fabricate lenses tailored to individual eye shapes and to incorporate drugs.36-41
Additionally, 3D printing allows for the integration of smart features, such as biosensors that monitor IOP or detect biomarkers for ocular diseases, offering potential breakthroughs in personalized eye care.37,42 As research advances, 3D-printed CLs could revolutionize ophthalmology by providing customized solutions that improve vision correction and diagnostic capabilities.
CLs for Imaging and Optical Enhancements
Smart CLs are poised to revolutionize AR and virtual reality technologies.1,43-45 Various companies are developing AR-enabled lenses that provide a seamless digital overlay within the wearer’s field of view. Review of the patent literature describes applications ranging from navigation aids to enhanced training environments. CLs also hold potential for low-vision rehab through magnifying optics, facial recognition, and zooming capabilities.1 By integrating mini cameras and motion sensors, these lenses could support visually impaired individuals in performing daily activities independently.
AR CLs are being further explored for military and surgical applications, in which heads-up displays provide real-time data without obstructing the wearer’s vision. However, challenges include ensuring lightweight power sources, heat dissipation, and user comfort.
Artificial Intelligence and CLs
Artificial intelligence (AI) enhances the functionality of CLs by analyzing collected data in real time. AI-driven platforms can evaluate meibography images for DED, assess tear film stability, analyze biomarkers, and predict corneal disease severity.46-52 This synergy between smart lenses and AI algorithms allows for personalized treatment and disease management. Furthermore, AI integration enables advanced fitting methods for keratoconus and orthokeratology lenses, predicting lens decentration and its impact on higher-order aberrations.53,54
As AI continues to evolve, it will play a pivotal role in automating diagnostic processes, improving patient outcomes, and advancing lens design.
Challenges and Future Directions
Potential applications of CLs are vast, but several challenges must be addressed to realize their full potential.3,43,55Biocompatibility and comfort remain critical to ensuring widespread adoption. Integrating complex electronics into soft materials poses additional technical hurdles, including power supply and data transmission. Regulatory approval processes are another bottleneck, requiring robust clinical trials to validate safety and efficacy. Collaboration between engineers, clinicians, material scientists, and regulatory agencies are essential to overcoming barriers. Novel applications need to be prescribed or they will be commercial failures.
In 2024, two highly innovative CLs—a photochromic lens and the first drug-delivering lens that delivered a topical antihistamine—were discontinued due to manufacturing challenges, regulatory hurdles in certain countries, and low adoption, despite years of development and significant investment.29,56
From diagnosing systemic and ocular diseases to delivering drugs and enhancing vision through AR, the possibilities are numerous and transformative. Advances in biomaterials, sensor technology, and AI are driving this evolution. By embracing innovations, clinicians can unlock the full potential of CLs.
References
1. Jones L, Hui A, Phan CM, et al. CLEAR - Contact lens technologies of the future. Cont Lens Anterior Eye. 2021;44;2:398-430. doi: 10.1016/j.clae.2021.02.007
2. Wu KY, Dave A, Carbonneau M, Tran SD. Smart contact lenses in ophthalmology: innovations, applications, and future prospects. Micromachines (Basel). 2024;15;7. doi: 10.3390/mi15070856
3. Liu X, Ye Y, et al. Smart contact lenses for healthcare monitoring and therapy. ACS Nano. 2024;18(9):6817-6844. doi: 10.1021/acsnano.3c12072
4. Vo DK, Trinh KTL. Advances in wearable biosensors for healthcare: current trends, applications, and future perspectives. Biosensors (Basel). 2024;14;11. doi: 10.3390/bios14110560
5. Perez AJ, Zeadally S. Recent advances in wearable sensing technologies. Sensors (Basel). 2021;21;20. doi: 10.3390/s21206828
6. Yuan M, Das R, McGlynn E, Ghannam R, Abbasi QH, Heidari H. Wireless communication and power harvesting in wearable contact lens sensors. IEEE Sensors Journal. 2021;21 12484-12497. Doi: 10.1109/JSEN.2021.3055077
7. Mirzajani H, Mirlou F, Istif E, Singh R, Beker L. Powering smart contact lenses for continuous health monitoring: recent advancements and future challenges. Biosens Bioelectron. 2022;197:113761. doi: 10.1016/j.bios.2021.113761
8. Yun J, Li Z, Miao X, et al. A tear-based battery charged by biofuel for smart contact lenses. Nano Energy. 2023;110:108344. doi: 10.1016/j.nanoen.2023.108344
9. Shetty KH, Desai DT, Patel HP, Shah DO, Willcox MDP, Maulvi F. Contact lens as an emerging platform for non-invasive bio-sensing: a review. Sensors and Actuators A: Physical. 2024;376:115617. doi: 10.1016/j.sna.2024.115617
10. Zhu Y, Li S, Li J, et al. Lab-on-a-contact lens: recent advances and future opportunities in diagnostics and therapeutics. Adv Mater. 2022;34(24):e2108389. doi: 10.1002/adma.202108389
11. Lane JD, Krumholz DM, Sack RA, Morris C. Tear glucose dynamics in diabetes mellitus. Curr Eye Res. 2006;31(11)895-901. doi: 10.1080/02713680600976552
12. Tummanapalli SS, Willcox MDP, et al. Tear film substance P: a potential biomarker for diabetic peripheral neuropathy. Ocul Surf. 2019;17(4):690-698. doi: 10.1016/j.jtos.2019.08.010
13. Mansouri K, Weinreb RN. Meeting an unmet need in glaucoma: continuous 24-h monitoring of intraocular pressure. Expert Rev Med Devices. 2012;9(3):225-231. doi: 10.1586/erd.12.14
14. Shioya S, Higashide T, Tsuchiya S, et al. Using 24-hr ocular dimensional profile recorded with a sensing contact lens to identify primary open-angle glaucoma patients with intraocular pressure constantly below the diagnostic threshold. Acta Ophthalmol. 2020;98(8):e1017-e1023. doi: 10.1111/aos.14453
15. Dunbar GE, Shen BY, Aref AA. The Sensimed Triggerfish contact lens sensor: efficacy, safety, and patient perspectives. Clin Ophthalmol. 2017;11:875-882. doi: 10.2147/OPTH.S109708
16. Chiou JC. The development of smart contact lens system: taking dry eye syndrome diagnosis as an example. Future Tech Expo 2019. futuretech.org.tw/futuretech/index.php?action=product_detail&prod_no=P0008700001856&web_lang=en-us
17. Moreddu R, Elsherif M, Butt H, Vigolo D, Yetisen AK. Contact lenses for continuous corneal temperature monitoring. RSC Adv. 2019;9(20):11433-11442. doi: 10.1039/c9ra00601j
18. Ho H, Parviz BA. Contact lens with integrated pulse oximeter. Google Patent #US8909311B2. 2014 Dec 9. patents.google.com/patent/US8909311B2/en
19. Jünemann A, Chorągiewicz T, Ozimek M, Grieb P, Rejdak R. Drug bioavailability from topically applied ocular drops. Does drop size matter? Ophthalmol J. 2016;1(1):29-35. doi: 10.5603/OJ.2016.0005
20. Lovrec-Krstic T, Orthaber K, Maver U, Sarenac T. Review of potential drug-eluting contact lens technologies. Materials (Basel). 2023;16;10. doi: 10.3390/ma16103653
21. Rykowska I, Nowak I, Nowak R.Soft contact lenses as drug delivery systems: a review. Molecules. 2021;26;18. doi: 10.3390/molecules26185577
22. Hui A, Boone A, Jones L. Uptake and release of ciprofloxacin-HCl from conventional and silicone hydrogel contact lens materials. Eye Contact Lens. 2008;34(5):266-271. doi: 10.1097/ICL.0b013e3181812ba2
23. Phan CM, Subbaraman LN, Jones L: In vitro uptake and release of natamycin from conventional and silicone hydrogel contact lens materials. Eye Contact Lens. 2013;39(2):162-168. doi: 10.1097/ICL.0b013e31827a7a07
24. Hui A, Willcox M. In vivo studies evaluating the use of contact lenses for drug delivery. Optom Vis Sci. 2016;93(4):367-376. doi: 10.1097/OPX.0000000000000809
25. Lanier OL, Christopher KG, Macoon RM, Yu Y, Sekar P, Chauhan A. Commercialization challenges for drug eluting contact lenses. Expert Opin Drug Deliv. 2020;17(8):1133-1149. doi: 10.1080/17425247.2020.1787983
26. Pollard TD, Seoane-Viano I, Ong JJ, et al. Inkjet drug printing onto contact lenses: Deposition optimisation and non-invasive dose verification. Int J Pharm X. 2023;5:100150. doi: 10.1016/j.ijpx.2022.100150
27. Polat HK, Kurt N, Aytekin E, Pehlivan SB, Çalış S. Novel drug delivery systems to improve the treatment of keratitis. J Ocul Pharmacol Ther. 2022;38(6):376-395. doi: 10.1089/jop.2021.0127
28. Chen X, Shen T. Advances in innovative delivery systems for antiglaucoma drugs. Curr Opin Ophthalmol. 2023; 34(2):123-128. doi: 10.1097/ICU.0000000000000933
29. Pall B, Gomes P, Yi F, Torkildsen G. Management of ocular allergy itch with an antihistamine-releasing contact lens. Cornea. 2019;38(6):713-717. doi: 10.1097/ICO.0000000000001911
30. Jacobs DS, Carrasquillo KG, Cottrell PD, et al. CLEAR - Medical use of contact lenses. Cont Lens Anterior Eye. 2021;44(2):289-329. doi: 10.1016/j.clae.2021.02.002
31. Nakhla M, Patel R, Crowley E, Li Y, Peiris TB, Brocks D. Utilizing PROSE as a drug delivery device for preservative-free cyclosporine 0.05% for the treatment of dry eye disease: a pilot study. Clin Ophthalmol. 2024;18:3203-3213. doi: 10.2147/OPTH.S487369
32. Yin J, Jacobs DS. Long-term outcome of using Prosthetic Replacement of Ocular Surface Ecosystem (PROSE) as a drug delivery system for bevacizumab in the treatment of corneal neovascularization. Ocul Surf. 2019;17(1):134-141. doi: 10.1016/j.jtos.2018.11.008
33. Novack GD, Barnett M. Ocular drug delivery systems using contact lenses. J Ocul Pharmacol Ther. 2020;36(8):595-601. doi: 10.1089/jop.2020.0024
34. Zhang X, Cao X, Qi P. Therapeutic contact lenses for ophthalmic drug delivery: major challenges. J Biomater Sci Polym Ed. 2020;31(4):549-560. doi: 10.1080/09205063.2020.1712175
35. Phan C-M. 3D printing of specialty contact lenses. Contact Lens Spectrum. 2023;38(8):43. clspectrum.com/issues/2023/august/fast-forward-to-the-future
36. Hisham M, Salih AE, Butt H. 3D printing of multimaterial contact lenses. ACS Biomater Sci Eng. 2023;9(7):4381-4391. doi: 10.1021/acsbiomaterials.3c00175
37. Alam F, Elsherif M, AlQattan B, et al. 3D printed contact lenses. ACS Biomater Sci Eng. 2021;7(2):794-803. doi: 10.1021/acsbiomaterials.0c01470
38. Barcena AJR, Dhal K, Patel P, Ravi P, Kundu S, Tappa K. Current biomedical applications of 3d-printed hydrogels. Gels. 2023;10(1):8. doi: 10.3390/gels10010008
39. Ganguly S, Wulff D, Phan C-M, Jones JW, Tang XS. Injectable and 3D extrusion printable hydrophilic silicone-based hydrogels for controlled ocular delivery of ophthalmic drugs. ACS Appl Bio Mater. 2024;7(9):6286-6296. doi: 10.1021/acsabm.4c00901
40. Mohamdeen YMG, Tabriz AG, Tighsazzadeh M, et al. Development of 3D printed drug-eluting contact lenses. J Pharm Pharmacol. 2022;74(10):1467-1476. doi: 10.1093/jpp/rgab173
41. Goto E, Tagami T, Ogawa K, Ozeki T. Fabrication of 3D-printed contact lens composed of polyethylene glycol diacrylate for controlled release of azithromycin. Biol Pharm Bull. 2023;46(10):1461-1467. doi: 10.1248/bpb.b23-00443
42. Sommer AC, Blumenthal EZ. Implementations of 3D printing in ophthalmology. Graefes Arch Clin Exp Ophthalmol. 2019;257(9):1815-1822. doi: 10.1007/s00417-019-04312-3
43. Efron N. Augmented reality contact lenses - so near yet so far. Clin Exp Optom 2023;106(4):349-350. doi: 10.1080/08164622.2023.2188176
44. Ko J, Kim G, Kim I, et al. Metasurface-embedded contact lenses for holographic light projection. Adv Sci (Weinh). 2024;11(38):e2407045. doi: 10.1002/advs.202407045
45. Fogt JS. Novel silicone elastomer contact lenses designed for simultaneous viewing of distance and near eye displays. Cont Lens Anterior Eye. 2023;46(4):101870. doi: 10.1016/j.clae.2023.101870
46. Graham AD, Kothapalli T, Wang J, et al. A machine learning approach to predicting dry eye-related signs, symptoms and diagnoses from meibography images. Heliyon. 2024;10(17):e36021. doi: 10.1016/j.heliyon.2024.e36021
47. Brahim I, Lamard M, Benyoussef A-A, Quellec G. Automation of dry eye disease quantitative assessment: A review. Clin Exp Ophthalmol. 2022;50(6):653-666. doi: 10.1111/ceo.14119
48. Ji Y, Liu S, Hong X, et al. Advances in artificial intelligence applications for ocular surface diseases diagnosis. Front Cell Dev Biol. 2022;10:1107689. doi: 10.3389/fcell.2022.1107689
49. Fineide F, Storas AM, Chen X, et al. Predicting an unstable tear film through artificial intelligence. Sci Rep. 2022;12(1):21416. doi: 10.1038/s41598-022-25821-y
50. Kang L, Ballouz D, Woodward MA. Artificial intelligence and corneal diseases. Curr Opin Ophthalmol. 2022;33(5):407-417. doi: 10.1097/ICU.0000000000000885
51. Yu Y, Zhou Y, Tian M, et al. Automatic identification of meibomian gland dysfunction with meibography images using deep learning. Int Ophthalmol. 2022;42(11):3275-3284. doi: 10.1007/s10792-022-02262-0
52. Pur DR, Krance SH, Pucchio A, Miranda RN, Feifeli T. Current uses of artificial intelligence in the analysis of biofluid markers involved in corneal and ocular surface diseases: a systematic review. Eye (Lond). 2023;37(10):2007-2019. doi: 10.1038/s41433-022-02307-9
53. Abadou J, Dahan S, Knoeri J, et al. Artificial intelligence versus conventional methods for RGP lens fitting in keratoconus. Cont Lens Anterior Eye. 2024;48(1):102321. doi: 10.1016/j.clae.2024.102321
54. Lopez Garcia Rosuero M, Arranz Bombin A, Romero R, Hornero R, Martin R. Clinical tool to measure fluorescein patterns in orthokeratology. PeerJ. 2022;10:e14068. doi: 10.7717/peerj.14068
55. Han F, Ge P, Wong F, et al. Smart contact Lenses: From rational design strategies to wearable health monitoring. Chem Eng J. 2024;497:154823. doi: 10.1016/j.cej.2024.154823
56. Renzi-Hammond LM, Buch JR, Hacker L, Cannon J, Hammond BR Jr.. The effect of a photochromic contact lens on visual function indoors: a randomized, controlled trial. Optom Vis Sci. 2020;97(7):526-530. doi: 10.1097/OPX.0000000000001537